PHOTONIC FRONTIERS: Linac Coherent Light Source begins operation
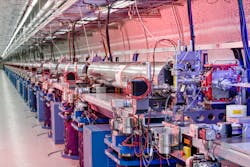
This month the SLAC National Accelerator Laboratory (Stanford, CA) will open the world's shortest-wavelength free-electron laser for use by guest scientists. First operated in April at 0.15 nm the Linac Coherent Light Source (LCLS) is the first free-electron laser to emit hard x-rays.1 The LCLS can deliver 80 fs pulses containing about 10 trillion x-ray photons at wavelengths tunable between about 0.15 and 1.5 nm (see Fig. 1).
"This is a landmark event in the history of light-source science, which will open up vast new areas for scientific exploration," writes Brian McNeil of the University of Strathcylde (Glasgow, Scotland).2 The combination of short pulse duration and short wavelength promise imaging of single atoms and molecules at a time resolution short enough to observe chemical processes.
For users, LCLS marks a huge increment in the brightness of hard-x-ray sources, a factor of 108 in terms that essentially measure peak power intensity per unit wavelength. For laser researchers, LCLS is an impressive demonstration of the capabilities of free-electron lasers.
FEL Background
Invented and first demonstrated by John M. J. Madey while he was at Stanford University (Stanford, CA) in the 1970s, the free-electron laser extracts energy from a beam of energetic electrons passing through a magnetic field that varies periodically in strength and direction along the path of the beam. The field comes from an array of magnets of alternating polarity called an undulator, and it bends the electrons' paths back and forth, causing them to release energy as light. The fundamental physics are the same as for synchrotron radiation when strong magnetic fields bend the paths of electrons (see Fig. 2).Free-electron lasers have been built to operate across a wide range of wavelengths by adjusting undulator spacing and electron energy. Producing hard x-rays requires electrons with very high energy, so LCLS was built to use the final kilometer of the existing two-mile Stanford Linear Accelerator, originally built in the 1960s for particle-physics research. That segment can accelerate electrons to 4.3 to 13.6 billion electron volts (GeV); changing electron energy tunes the wavelength.
The undulator magnet period is three centimeters, with a total of about 112 m of magnets stretching along 132 m of the beam transport line, says John Galayda, director of LCLS construction. The undulators are assembled in 3.4 m modules, spaced about 60 cm apart along the transport line. Mechanical and magnetic field tolerances are tight to assure proper operation.
Successful operation at hard-x-ray wavelengths requires sustaining a delicate balance in interactions among the electrons and photons, so the electrons remain tightly bunched and interact coherently with the light that propagates along with them. In laser terms, this means keeping the electrons tightly bunched so the stimulated emission is coherent with the original spontaneously emitted photons. The result is self-amplified stimulated emission, which eventually saturates as propagating electrons begin to disperse. The dispersion is inevitable, but the goal is to keep the electrons bunched through the undulator, generating an intense coherent pulse lasting on the order of 100 fs. The coherence comes from the tight clumping of the emitting electrons; LCLS is a single-pass device without a resonator.
The LCLS segment of the SLAC accelerator delivers peak currents of 1000 to 3000 A in intense ultrashort pulses. The electron pulse must be kept tightly concentrated so the electrons are bathed in their own light emission. This requires keeping the e-beam size comparable to that of the x-ray beam, and keeping its path straight to within several micrometers through the entire 132 m of undulators.
Elaborate and exacting controls are needed because the tolerances scale with the wavelength, and are thus far tighter than for other free-electron lasers. "We have to compensate for the Earth's magnetic field and account for ground settlement, which can accumulate over a few weeks," says Galayda. Alignment is accomplished with precision motorized systems.
"We scared ourselves"
Goals of the LCLS are production of 78 fs pulses containing a trillion (1012) 0.15 nm photons, each with 8 keV of energy. Each pulse delivers more than a millijoule of energy, with peak power of about 10 GW. Early test runs were at repetition rates of 10 Hz, but when testing is finished LCLS is designed to run at the linear accelerator's standard rate of 120 Hz.
To achieve those ambitious goals, SLAC began characterizing the electron beam in 2007, doing extensive work to be sure it reached the desired quality and was in the proper place before installing the first undulators. In April they had installed 20 of the 33 undulator modules, enough for the laser to operate. "The power increased exponentially, as we had hoped, with no steps backwards, and the FEL operated the first time we tried to lase," recalled Galayda. He had worked on other FELs that had teething problems, so he didn't expect LCLS to be easy. Starting up what he called "the most difficult light source that has ever been turned on" took only two hours. "We scared ourselves. Usually there's something miswired, but every last clip was hooked up right." Since then, Galayda has been tuning up LCLS to get ready for regular operation for guest scientists.
The free-electron laser now has full use of its part of the linear accelerator. The upstream segment of the accelerator can be used independently for other experiments, but LCLS won't have to share its part.
User facility instruments
As is common for x-ray sources serving a broad community of users, output from LCLS will be switched among several sets of instruments with different capabilities. These include:
•Atomic, molecular and optical science instruments including focusing optics, gas delivery system, a high-power laser that can be synchronized with LCLS, and electron, ion, and photon spectrometers.
•An x-ray correlation spectroscopy instrument to study materials either on time scales from 100 fs to several nanoseconds, or from 10 ms to thousands of seconds.
•An x-ray pump-probe instrument in which a short-pulse optical laser pumps target materials to short-lived states that are probed by observing scattering of LCLS X-ray pulses.
•A coherent x-ray imaging instrument to record images of single particles illuminated by pulses from LCLS, including nanoparticles and single biomolecules.
•Soft x-ray materials-science instruments including a high-resolution monochromator for spectroscopy at photon energies between 500 and 2000 eV, and optics to allow use of other equipment.
•A proposed matter in extreme conditions instrument will be designed to observe matter at temperatures above 10,000 degrees Kelvin and pressures about 10 million atmospheres.
X-ray lasers and synchrotrons
X-rays became powerful tools for studying atomic and solid-state physics after early twentieth-century physicists realized crystals scatter x-rays like a diffraction grating scatters light. But the nature of x-ray sources has always limited the information that they could gather from matter.
Today's standard source of hard x-rays is synchrotron radiation, discovered in the 1940s. Synchrotrons can generate a wide range of wavelengths and higher intensities than conventional x-ray tubes. Yet synchrotrons are big and expensive, normally operated as user facilities such as the Stanford Synchrotron Radiation Laboratory. Also, synchrotron radiation is broadband, more like white light than a laser. X-ray lasing has been demonstrated in laser-produced plasmas since the 1980s, but reaching short wavelengths has required extremely powerful lasers with very low repetition rates, making experiments difficult.
X-ray free-electron lasers are big and costly, but they operate at reasonable repetition rates, making them suitable for national or regional user facilities. The first one, the Free-electron LASer at Hamburg (FLASH) was built to use an existing accelerator at the Deutsches Elektronen-Synchrotron (DESY; Hamburg, Germany). Turned on three years ago, it initially emitted at 13.5 nm, and has since been upgraded to 6.5 nm.3
The LCLS also uses an existing accelerator, but takes a big step to more energetic x-rays at much higher brightness than ever before available. "It's basically an x-ray wavelength strobe" that can take snapshots of extremely short pulses on extremely small scales, says Galayda. During its 80 fs pulse, an atom normally moves only about one atomic diameter, so the x-ray pulses should show atoms dissociating from molecules, and observe chemical reactions and shock-waves (see Fig. 3).Researchers also are excited by prospects for determining the spacing and orientations of atoms in nanoparticles or single biomolecules. Galayda envisions dropping single protein molecules one by one, zapping each one with an x-ray pulse, and overlapping the resulting data to reconstruct the structure of the molecules. Conventional synchrotron-source x-ray crystallography can do that for crystalline materials, but many of the most interesting proteins are extremely difficult to crystallize. Using LCLS to zap single proteins from cell membranes could take years off the time needed to determine their structure by conventional means that require solving difficult problems to grow large enough crystals.
Those are only the obvious examples, says Galayda. "We're all expecting that the first users will come up with new ideas" for using LCLS. With other x-ray free-electron lasers under construction in Japan (SCSS) and Germany (XFEL) we can expect even more ideas to follow.
REFERENCES
1. http://lcls.slac.stanford.edu/
2. Brian McNeil, "First light from hard X-ray laser," Nature Photonics 3, pp. 375 (Jul 2009).
3. http://hasylab.desy.de/facilities/flash/index_eng.html
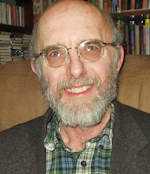
Jeff Hecht | Contributing Editor
Jeff Hecht is a regular contributing editor to Laser Focus World and has been covering the laser industry for 35 years. A prolific book author, Jeff's published works include “Understanding Fiber Optics,” “Understanding Lasers,” “The Laser Guidebook,” and “Beam Weapons: The Next Arms Race.” He also has written books on the histories of lasers and fiber optics, including “City of Light: The Story of Fiber Optics,” and “Beam: The Race to Make the Laser.” Find out more at jeffhecht.com.