Scientists strongly couple light to a micromechanical object
Physicists at the Institute for Quantum Optics and Quantum Information (IQOQI; Vienna and Innsbruck, Austria) have created an interaction between photons and a micromechanical resonator that is strong enough to transfer quantum effects.1 This is an important step towards quantum physics experiments in the macroscopic domain.
Quantum physics is full of paradoxes that are in conflict with everyday experience. Do the laws of quantum physics apply to "everyday" objects visible to the naked eye? Such a question has been posed by physicists like Erwin Schrödinger since the beginnings of quantum theory. With today's nano- and microfabrication capabilities such experiments are within reach.
Researchers worldwide have begun to look into quantum experimentation with mechanically oscillating objects. Such mechanical resonators can vary in size from a few hundred nanometers up to several centimeters and would therefore constitute by far the biggest objects on which quantum theory has been tested.
One approach is to transfer the properties of an elementary quantum system--for example, a single electron, atom, or photon--onto the macroscopic mechanical object. However, two conditions have to be met: first, the mechanical resonator has to be cooled down to temperatures close to absolute zero; and second, the force between the mechanical resonator and the electron, atom, or photon has to be strong enough to overcome the natural decay of the quantum properties, or the so-called decoherence. Thus far, neither of these conditions has been fulfilled.
Now, Markus Aspelmeyer and other researchers at IQOQI have demonstrated the second requirement for observing quantum effects: the "strong coupling" regime between a mechanical object and photons.
Coupled motion of light and mechanics
Aspelmeyer's group used a mechanical bridge for the experiments: with a width of 50 microns and a length of 150 microns, the bridge is visible to the naked eye. A mirror with a 50 micron diameter is attached to the mechanical bridge such that photons hitting the mirror are reflected and exert a force onto the bridge
"This is the same radiation-pressure force that we already used in 2006 to demonstrate mechanical laser-cooling," says Aspelmeyer. "To generate the desired strong coupling, we use a well-established method from quantum optics: an optical resonator. Because a single reflection of a photon does not exert a sufficiently large force, we reflect the light back and forth between the small mirror and a second, larger mirror, thereby multiplying the force until the photon escapes through one of the two mirrors due to their imperfect reflectivity."
If the number of photons in the light beam is too small, however, it still takes too long for the force between the light and the mechanics to build up. In this case, decoherence dominates and the light field between the two mirrors oscillates essentially independently of the mechanical motion of the bridge. "For large laser intensities, however, the situation changes dramatically: the energy exchange between the light and the mechanics happens faster than the time the photons need to exit the optical resonator and hence the motion of the light and the mechanics becomes coupled," explains Aspelmeyer.
An optomechanical pendulum
"The situation is analogous to two pendulums, for example two grandfather clocks, that are coupled either via a soft rubber band or via a stiff spring, notes Aspelmeyer. "In the first case," he says, "the pendulums swing independently of each other, whereas in the second case, the two systems exhibit a completely new, characteristic oscillation pattern due to the 'strong coupling.'"
The experiment is the first to show this effect between a massive mechanical pendulum and an optical light field. Up to now, this was only possible in the size domain of a few atoms or very small quantum systems. The generated oscillations are neither purely optical nor purely mechanical, but rather a true hybrid optomechanical excitation, a feature of particular interest for future quantum experiments.
"We have clearly found the oscillation pattern of the strongly coupled 'optomechanical' pendulum in the energy spectrum of the light leaking out of the optical resonator," Aspelmeyer adds.
After this step, the researchers now hope that, with the help of additional cooling like the previously successfully implemented mechanical laser-cooling, they can soon observe quantum behavior of mechanical objects. "The next goal is to combine the strong coupling with the cooling of the mechanics," says Simon Gröblacher, a Ph.D. student in Aspelmeyer's team. "With this experiment, we are on the cusp of being able to test how far into our macroscopic world the laws of quantum physics are valid."
The research results are the outcome of a fruitful collaboration between experimental and theoretical physicists of the Institute for Quantum Optics and Quantum Information: the theoretician Klemens Hammerer from Innsbruck supported the Vienna team lead by Markus Aspelmeyer with the theory of the experiment and the interpretation of the data. The researchers were supported by the Austrian Science Fund, the European Commission, and the Foundational Questions Institute.
For more information, contact Markus Aspelmeyer at: [email protected]
REFERENCE
1. S. Gröblacher et al., Nature 460, p. 724 (6 August 2009), http://dx.doi.org/10.1038/nature08171
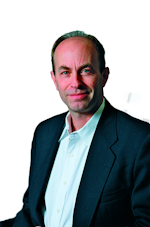
John Wallace | Senior Technical Editor (1998-2022)
John Wallace was with Laser Focus World for nearly 25 years, retiring in late June 2022. He obtained a bachelor's degree in mechanical engineering and physics at Rutgers University and a master's in optical engineering at the University of Rochester. Before becoming an editor, John worked as an engineer at RCA, Exxon, Eastman Kodak, and GCA Corporation.