PRODUCT FOCUS: INTEGRATING SPHERES: How to select an integrating sphere for your application
Integrating spheres seem straightforward: optical devices comprising a hollow spherical cavity coated on the inside with a special highly reflective Lambertian coating used to uniformly scatter and diffuse incident light, with entrance and exit ports. How complicated can it be to find the right one for your application? Very. The choices of possible configurations are infinite, as varied as the applications that use them (see Fig. 1). We spoke to manufacturers of integrating spheres for some directions on the complicated road to finding the right sphere for a particular application.
First, it helps to understand how integrating spheres work and when they are needed. An integrating sphere (IS) acts like a diffuser, preserving power but neglecting spatial information. Light entering the sphere undergoes multiple scattering reflections, so that rays that are incident on any point on the inner surface are equally distributed, minimizing the effects of the original direction of the rays.
“Typically integrating spheres are used when light, whether it’s emitted, reflected, or transmitted, is scattered and one wants to catch the most possible light,” says Art Springsteen, president of Avian Technologies (Sunapee, NH). For diffuse reflectance, transmittance, and scattering measurements in spectroscopy (as in turbidity), an integrating sphere is the best choice. Spheres are also used to measure total luminous flux and total spectral radiance.
When should you use an IS rather than a spectrometer or power meter? A sphere has a couple of major advantages over conventional power meters, explains Chris Durell, vice president, sales and applications engineering, Sphereoptics (Concord, NH). “The first is uniform response independent of spatial and angular information. Spheres do not care about the angular profile of the source and spatial distribution—only input power.” This is useful for measurements of diodes or fibers with angular divergence that impinges on the quality of the power measurement. “On the other hand,” says Durell, “if you want beam profiles and angular information, then use a beam profiler or goniometer. A sphere will, by definition, wipe out this information.”
The second major advantage of a sphere over power meters is attenuation. “The sphere is a uniform attenuator. It can be used in power measurements where a conventional meter might be damaged by the power level of the source,” says Durell. This can also be a negative as spheres are significantly less sensitive than conventional power meters. “In sphere-based power measurement, 1 µW is a typical threshold for good signal-to-noise ratio, versus 1 nW or less for conventional power meters.” In addition to capturing all the light from any light source, an IS can be calibrated to NIST-traceable standards to measure spectral flux.
What’s your app?
Integrating spheres are used in numerous applications, such as optical power measurement of light sources and lasers, and in spectral and spectral-power-density measurements of light-emitting diodes (LEDs). They are also used to measure reflection from and transmission through samples. The spheres can also be used to generate a uniform field of light to calibrate remote-sensing cameras.
Manufacturers agree that the complex process of fitting an IS to a customer begins with the details of their application. “The nuances of how applications affect design has kept me on a learning curve for 15 years, and still provides challenges today,” says Durell at Sphereoptics. Integrating-sphere applications fit into four basic categories: uniform sources, lamp or light measurements, reflectance and transmittance, and laser power measurements. “Each category has a variety of solutions and nuanced subapplications that require changing the spheres in subtle ways to provide optimal performance,” says Durell.
The two most common applications for integrating spheres are as measuring tools to measure the total flux of lamps, and as uniform light sources to calibrate other instruments, according to Bob Angelo, president, Gigahertz-Optik (Newburyport, MA), a subsidiary of Gigahertz-Optik GmbH (Puchheim, Germany). And in those two categories, integrating spheres are particularly versatile, able to integrate light from sources that emit in a narrow collimated beam, such as a laser, or from omnidirectional sources like an incandescent bulb or fluorescent tube.
Size matters
Beyond the four basic categories, integrating devices can be categorized further by size and coating. Sphere size is measured by the inside diameter, and can range from 1 mm up to 3 m. A very small sphere can be required simply because it may need to fit inside another device (see Fig. 2). In the case of a fast-pulsed laser-power measurement, says Angelo, a detector using a small IS will ensure that detection rise time is not adversely effected. For very large multidirectional light sources like high-pressure sodium lamps or long fluorescent tubes, an IS larger than 1 m in diameter can be required to fit and center the lamp inside the sphere. A large diameter might also be required if the sphere is used as a light source to provide a large, uniform, light-exposure field.Greg McKee, director, systems business unit at Labsphere—the first to manufacture spheres in the US—gives several main factors that determine the minimum size of a sphere: the physical dimensions of the lamp to be measured (leaving room for the baffle), the self-absorption of the lamp, and the internal sphere temperature. The lamp mounting hardware must also fit inside. On the other hand, the sphere must not be too big or its responsivity is limited: The diameter is ideally ten times the largest dimension of a small lamp, or twice the length of a long lamp being measured. Practically, 1-to-3-m spheres are used for luminous-flux measurements of the most common incandescent and compact fluorescent lamps. Spheres of 2 m or more are typically used to measure light sources of 500 W or larger.
Most spheres are made of lightweight aluminum, but other materials such as steel, plastic, and fiberglass have been used. “It’s hard to make spheres physically uniform, which is the crux of producing a uniform distribution of light,” says Alex Fong at Optronic Laboratories (Orlando, FL). Joining the two halves or quadrants and managing the sealing/seams is also easiest with aluminum. “People have tried just painting a large room to act as integrating spheres, but aluminum is the best material so far.”
Coatings
Perhaps the most important parameter to determine when selecting an integrating sphere is the reflectivity of the coating or material—the higher the better. “Higher reflectivity means light has more reflections inside the sphere before it is absorbed,” says Peter Weitzman, vice president, sales and marketing, Labsphere, “therefore the integration is better and the measurement accuracy better.”
Coatings, which can be spray-on or a powder, are dependent on the environmental conditions and, more important, the wavelength range over which the sphere is used. “You want as uniform a performance as possible across the entire spectral range of interest, which is why they tend to be white,” says Fong. “Coatings mark one of the most important proprietary differences between manufacturers.”
Typical spray-on reflective coatings are barium sulfate (BaSO4)-based with a binder, which are primarily used in the visible region, though they can be stretched for use in the near-ultraviolet and the near-infrared (NIR). For NIR to mid-IR applications, diffuse gold coatings are typically used. In extreme conditions or for small spheres, sintered polytetrafluoroethylene (PTFE or Teflon) gives the best performance. “It’s an ideal material—except for the weight (heavy) and cost (high),” says Springsteen. Manufacturers often publish an overview of each coating they offer and their optimal wavelength range on their web site.
Configurations
Beyond size and coating, sphere configuration is one of the most challenging parameters to determine. How many entrance and detector ports will you need? Some configurations can require as many as 18 ports. Will you need a baffle, and if so, how big? Baffles prevent direct source light from saturating or damaging the detector and must be as small as possible to minimize shadows. In high-divergence laser-diode measurements, baffles can be eliminated, and the detector moved closer to the entrance port to eliminate that first hot spot of light and minimize the possibility of saturating or damaging the photodiode.Other key specifications to understand are the unit of measurement you want to acquire (power in W, irradiance in W/m2, or luminous flux in lumens), and the geometry of your sphere, whether full-sphere 4π steradians or hemisphere 2π (see Fig. 3). “A full sphere can measure devices that emit in all directions (4π steradians) as well as forward-emitting only (2π),” says Weitzman. “A half sphere is typically only used for 2π measurements.” The right manufacturer welcomes a personal consultation to figure out the correct design for you.
Editor’s note: The “Product Focus” series is intended to provide a broad overview of the product types discussed. Laser Focus World does not endorse or recommend any of the products mentioned in this article.
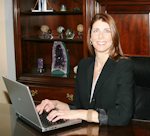
Valerie Coffey-Rosich | Contributing Editor
Valerie Coffey-Rosich is a freelance science and technology writer and editor and a contributing editor for Laser Focus World; she previously served as an Associate Technical Editor (2000-2003) and a Senior Technical Editor (2007-2008) for Laser Focus World.
Valerie holds a BS in physics from the University of Nevada, Reno, and an MA in astronomy from Boston University. She specializes in editing and writing about optics, photonics, astronomy, and physics in academic, reference, and business-to-business publications. In addition to Laser Focus World, her work has appeared online and in print for clients such as the American Institute of Physics, American Heritage Dictionary, BioPhotonics, Encyclopedia Britannica, EuroPhotonics, the Optical Society of America, Photonics Focus, Photonics Spectra, Sky & Telescope, and many others. She is based in Palm Springs, California.