Caltech silicon micromechanical system produces squeezed light, useful for ultraprecise interferometry
Pasadena, CA--"Squeezed light" is a nonclassical state of light in which the amount of quantum noise depends on the phase (or other characteristic) of the light wave; in some cases, optical interferometers can measure distances more precisely when relying on squeezed light than when using ordinary light.
LIGO wants squeezed light
As a result, the Laser Interferometer Gravitational-Wave Observatory (LIGO) scientific collaboration has invested heavily in research on squeezed light because of its potential to enhance the sensitivity of gravitational-wave detectors. Squeezed light has been created using nonlinear optical crystals and other techniques. Now, in the latest approach, a team led by researchers at the California Institute of Technology (Caltech) has engineered a miniature silicon system that produces squeezed light through micromechanical means. The system is miniaturized on a silicon microchip, generating the ultra-quiet light in a way that can be more easily adapted to a variety of sensor applications.
In the device, light is coupled into the device via a narrow waveguide and reflects off a back mirror formed by a linear array of etched holes (see figure). Upon reflection, the light interacts with a pair of double nanobeams (micromechanical resonator/optical cavity), which are deflected in a way that tends to cancel fluctuations in the light.
"This system should enable a new set of precision microsensors capable of beating standard limits set by quantum mechanics," says Oskar Painter, a professor of applied physics at Caltech and the senior author of a paper that describes the system; the paper appears in the August 8 issue of the journal Nature. "Our experiment brings together, in a tiny microchip package, many aspects of work that has been done in quantum optics and precision measurement over the last 40 years."
"We work with a material that's very plain in terms of its optical properties," says Amir Safavi-Naeini, a graduate student in Painter's group and one of three lead authors of the paper. "We make it special by engineering or punching holes into it, making these mechanical structures that respond to light in a very novel way. Of course, silicon is also a material that is technologically very amenable to fabrication and integration, enabling a great many applications in electronics."
Interaction of light with silicon beams cancels noise
In this new system, a waveguide feeds laser light into a cavity created by two silicon beams. Once there, the light bounces back and forth a bit thanks to the engineered holes, which effectively turn the beams into mirrors. The photons cause the beams to vibrate; the particulate nature of the light introduces quantum fluctuations that affect those vibrations. The light and beams interact so strongly with each other that the beams impart the quantum fluctuations they experience back on the light. And, as is the case with the noise-canceling technology used, for example, in some headphones, the fluctuations that shake the beams interfere with the fluctuations of the light. They effectively cancel each other out, eliminating the noise in the light.
In the experiment, a detector measuring the noise in the light as a function of frequency showed that in a frequency range centered around 28 MHz, the system produces light with less noise than what is present in a vacuum—the standard quantum limit. However, the structure could be redesigned to create squeezed light at other frequencies. Many signals are specific to a particular frequency range; for example, in the case of LIGO, some frequencies are intimately related to the dynamics of astrophysical objects such as circling black holes. Because the optical squeezing occurs near the mechanical resonance frequency where an individual device is most sensitive to external forces, this feature would enable the system studied by the Caltech team to be optimized for targeting specific signals.
For more info, see https://www.caltech.edu/about/news/caltech-team-produces-squeezed-light-using-silicon-micromechanical-system-40014
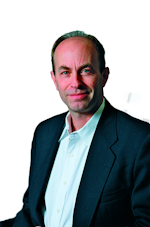
John Wallace | Senior Technical Editor (1998-2022)
John Wallace was with Laser Focus World for nearly 25 years, retiring in late June 2022. He obtained a bachelor's degree in mechanical engineering and physics at Rutgers University and a master's in optical engineering at the University of Rochester. Before becoming an editor, John worked as an engineer at RCA, Exxon, Eastman Kodak, and GCA Corporation.