PHOTONIC FRONTIERS: ADAPTIVE OPTICS: Adapting adaptive optics to aid human vision
The use of adaptive optics was initiated by efforts to develop high-energy laser weapons. The powerful beams of the lasers perturbed the atmosphere, prompting developers to devise adaptive optical systems to compensate for those perturbations and deliver more laser power to the target. Astronomers quickly realized that adaptive optics could improve seeing through the turbulent atmosphere, which otherwise limits even the best ground-based telescopes to resolution far below the diffraction limit imposed by their size. Although limited to imaging small regions at one time, adaptive optics have been an impressive astronomical success.
FIGURE 1. Conventional corrective lenses only compensate for defocus and astigmatism in the eye. Adaptive optics can also measure higher-order aberrations, which are small in most people but impair the vision of a minority of people.
Now adaptive optics are compensating for perturbations in a different transmission medium-the human eye. Some adaptive-optic systems are already used in research, most often to clearly image individual retinal cells, which are normally blurred by the imperfections of the human eye. Others are being developed for diagnosis of eye problems, such as an automated adaptive-optic system that can measure the optical imperfections of the living eye to prescribe corrective lenses. There’s even talk of developing adaptive lenses for use either as contact lenses or implants, but that is likely to be a long way off.
The imperfect eye
The human eye is far from a perfect optical instrument. Standard corrective lenses can compensate for defocusing on the retina and astigmatism, but not for higher-order aberrations such as coma and spherical aberration (see Fig. 1). For most people, these high-order aberrations have little practical impact on vision, but they do perturb the wavefront of light enough to limit how well optical instruments can see inside the eye for medical research and diagnosis.
Adaptive optics can sense and compensate for these higher-order aberrations, improving the resolution of optical instruments so they can routinely see individual cells in the retina. Like other adaptive-optic systems, those for examining the eye direct a probe beam into the eye, analyze the perturbed wavefront with a wavefront sensor, then process signals from the sensor to change the shape of an adaptive mirror so it compensates for the perturbations. This feedback loop effectively damps out the perturbations, improving image quality (see Fig. 2).
Retinal imaging
Under unusual circumstances, conventional optics can compensate for the aberrations in the eye well enough so that a fundus camera can barely resolve single retinal cells. However, adaptive optics can attain far better resolution, particularly when observing the cones that provide detail and color vision (see Fig. 3). As Brian O’Brien recognized a half-century ago at the University of Rochester, cones act like tiny optical fibers aligned toward the pupil, guiding light along their length until the pigmented part of the retinal cell reflects the light back toward the pupil. This efficient reflection makes wavefront compensation possible even with low levels of illuminating light, so adaptive optical systems can easily resolve individual retinal cells.
FIGURE 3. Individual retinal cells are blurred with conventional imaging (left), but are clearly visible with adaptive optics (right). Both images are the averaged sums of six individual images.
This high resolution opened new doors for research. Color-vision researchers have known that only 5% of the cones had short-wavelength blue receptors. However, they could not tell the distribution of color receptors because they couldn’t resolve individual cones. At Rochester, Austin Roorda and David Williams used adaptive optics to identify the receptors present in individual cones, and showed that the ratio of green to red receptors varied widely among individuals without affecting color discrimination.1 They also found that the axes of the cones were aligned with remarkable precision toward a spot on the pupil just 150 µm across.
Improving instruments that measure the eye
Adaptive-optic techniques also can improve performance of conventional eye-measurement instruments. One is the scanning laser ophthalmoscope, which raster-scans a low-power laser beam across the retina to build up an image. Confocal imaging increases image contrast, and by adjusting the focal length can record images at different depths within the retina. Adding an adaptive optical system with dynamic compensation allowed Roorda, now at the University of California at Berkeley, to improve lateral and axial resolution, and to image blood flow through capillaries.2 With wavefront correction, the scanning system can also write extremely sharp images onto the retina, enabling the study of how retinal anatomy and neural processing affect vision.
Optical coherence tomography can’t match the lateral resolution of other techniques, but it has much better axial resolution-about 10 µm in the lab and 25 µm in commercial instruments. Addition of a closed-loop adaptive-optics system improved lateral resolution to between 5 and 10 µm and axial resolution to 3 µm, a significant improvement but not yet enough to resolve key structures such as photoreceptors and capillaries.3
The instrument most familiar to the public at large is the phoropter, the binocular device containing dozens of lenses used to test vision. Sometimes called a “refractor,” the phoropter is a time-tested tool for prescribing corrective lenses, but can be frustrating for patients asked to assess how much swapping lenses changes their view of a test screen. Autorefractors have been developed that use conventional optics to measure the eye’s optical properties, but they are only accurate enough to make a first approximation of the required correction. A precise prescription still requires a phoropter.
Developers are working on adaptive-optics systems that could replace the venerable mechanical phoropter and the autorefractor. The idea is to use a wavefront sensor to measure the aberrations in the patient’s eyes, and then adjust the surface of an adaptive mirror to correct the visual flaws. The patient then could check the correction by viewing images with the compensated mirrors. As with a mechanical phoropter, the optometrist or ophthalmologist could tweak the correction to the patient’s preference, testing by changing the correction applied to the adaptive mirror. An emerging generation of wavefront sensors will be able to measure all the properties of the eye very accurately, Roorda says. Several companies are working on the next challenge, developing software to convert measured aberrations into a prescription for a corrective lens.
‘Super vision’ and refractive surgery
Ophthalmologists already use wavefront sensors to develop custom corrections for patients undergoing refractive surgery, but the main goal usually has been to achieve nominally perfect 20/20 vision. A more ambitious and controversial idea, originally suggested by Williams and two colleagues in 1997, is to use adaptive optics to measure higher-order aberrations, and correct them to provide “super vision” more acute than is possible normally.4
This idea excited the refractive-surgery community, which saw a potentially lucrative new market in improving vision beyond what was once considered perfection. Williams now worries that the potential of surgical correction for higher-order aberrations is being overplayed for most people. The technique is attractive for people with severe higher-order aberrations that are otherwise hard to correct. “If you enter the office with a lot of higher-order aberrations, this customized vision correction can reduce those aberrations and give you a better result,” he says. However, he warns that the refractive surgery also introduces new aberrations, so patients who start with only small high-order aberrations will likely have stronger aberrations after surgery.
Adaptive-optic vision
The ultimate step may be to develop adaptive-optic lenses that could be worn as contact lenses or implanted in the eye to correct for eye aberrations and change their focal length to enhance depth adaptation and avoid the need for bifocals. Two years ago, Gleb Vdovin of the Technical University of Delft demonstrated a liquid-crystal wavefront corrector with 5‑mm aperture that could adjust its corrective power between 0 and +3 diopters.5 In principle, a wireless link might connect it to a wavefront sensor to correct vision.
It may sound tempting, but Roorda cautions “it’s a long way off.” Major problems include making the precision adjustments in lens shape needed for dynamic wavefront correction. For the foreseeable future, we’ll have to settle for using adaptive optics to make better static corrections to our vision.❏
REFERENCES
1. A. Roorda and D. R. Williams, Nature 397, 520 (1999).
2. A. Roorda et al, Optics Express 10, 405 (May 6, 2002).
3. B. Hermann et al, Optics Letters 29, 2142 (2004).
4. J. Liang. D. R. Williams, and D. T. Miller, J. Optical Society of America A 14, 2884 (1997).
5. G. Vdovin et al, Optics Express 11, 810 (April 7, 2003).
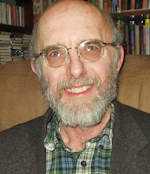
Jeff Hecht | Contributing Editor
Jeff Hecht is a regular contributing editor to Laser Focus World and has been covering the laser industry for 35 years. A prolific book author, Jeff's published works include “Understanding Fiber Optics,” “Understanding Lasers,” “The Laser Guidebook,” and “Beam Weapons: The Next Arms Race.” He also has written books on the histories of lasers and fiber optics, including “City of Light: The Story of Fiber Optics,” and “Beam: The Race to Make the Laser.” Find out more at jeffhecht.com.