OPTICAL MATERIALS: Laser pyrolysis produces photonic nanomaterials
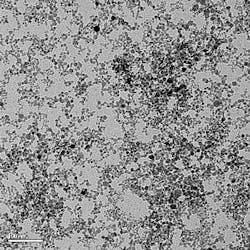
Laser pyrolysis is a gas-phase process in which light at 10.6 µm from a continuous-wave carbon dioxide (CO2) laser is absorbed by a material in a flowing gas stream; the absorbed energy is then transferred to non-laser-active molecules in the stream. The end product, at least in a version of the process developed by researchers at NanoGram (San Jose, CA), is a range of nanoscale particles with great relevance to photonics. The resulting materials can be used as phosphors for solid-state lighting, high-refractive-index fillers for optical polymer composites, thermochromic materials, and doped-glass films for planar photonics.
There is more than one way to carry out laser pyrolysis (which can include photolysis, or light-initiated molecular dissociation, as well). First, precursor materials can react or decompose to form nanoparticles. Second, sensitizers can cause other materials to decompose. And finally, substances can decompose, triggering the decomposition of other substances. In any case, the reactants are subsequently quenched at a controlled time to form nanoparticles, and then the particles collected.
High-index composite
In one example, high-index inorganic nanoparticles are created for use in transparent polymers; the particles, which are less than 20 nm in size, raise the index of the polymer while keeping transmission high and scattering low. Nanosize particles of rutile, a form of titanium dioxide (TiO2), can be made from vaporized titanium tetrachloride, with ethylene and oxygen as reactants. Nanogram’s process produces rutile particles with a uniform average particle size smaller than 10 nm (see figure).
The conventional processes of flame pyrolysis and sol-gel synthesis tend to produce nanoparticles of TiO2 in the less-desirable anatase form (which is photocatalytic), rather than the more-stable rutile. In general, nanoparticles with a uniform size of anywhere between 10 and 200 nm can be created.
High-index polymer nanocomposites are useful as transparent packaging for light-emitting diodes (LEDs) because the composites allow more light to be extracted from the LEDs, boosting their efficiency. NanoGram researchers have developed highly transparent nanocomposite films in which the 1.50 refractive index of the polymer itself is raised to 1.80. The films exhibit transmissions of well over 90% and are highly uniform.
Nanocomposites can also be used to improve the properties of microstructured optics. “NanoGram has demonstrated an optical reflector (a 2-D photonic crystal) that is a multilayer stack of high-index (nanocomposite) and low-index polymer films,” says Shiv Chiruvolu, one of the researchers. “In addition, already demonstrated using this technology are high-quality optical waveguide devices (fabricated using silicate glass deposition by laser pyrolysis-based laser-reactive deposition) with an optical loss of less than 0.1 dB/cm.”
Although the process is subatmospheric, high vacuum is not required. Pilot production is being scaled to a production rate of tens to hundreds of grams per hour; NanoGram believes the process can be further scaled to an output of greater than 100 tons per year. The stream of quenched nanoparticles from the process can be deposited directly on substrates with a single-pass deposition thickness of up to 30 µm on substrates up to 200 mm square. “We are currently working on developing applications with partner companies,” says Chiruvolu.
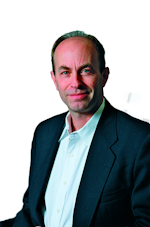
John Wallace | Senior Technical Editor (1998-2022)
John Wallace was with Laser Focus World for nearly 25 years, retiring in late June 2022. He obtained a bachelor's degree in mechanical engineering and physics at Rutgers University and a master's in optical engineering at the University of Rochester. Before becoming an editor, John worked as an engineer at RCA, Exxon, Eastman Kodak, and GCA Corporation.