PHOTONIC FRONTIERS: SINGLE-PHOTON LIGHT SOURCES: It’s hard to get just one photon
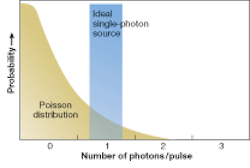
Quantum information systems promise a wonderland of opportunities. The best publicized is quantum cryptography, in which the laws of quantum mechanics promise the ultimate in data security. Send a single photon between two points, and any attempt to measure its properties anywhere along the route will alter its quantum state, revealing the presence of an eavesdropper (see www.laserfocusworld.com/articles/255503). Quantum computing and quantum communications likewise promise to improve computing and communications capabilities.
Realizing those quantum possibilities, however, requires a light source capable of generating exactly one photon at a time. Adding a second photon to a pulse punches a loophole in quantum cryptography because an eavesdropper can examine one photon but let the second pass unaltered to its destination-leaving no trace of the spy. Quantum information processing requires indistinguishable photons, identical in frequency and without a phase jump, so they can interfere with each other. And the light source should be efficient, emitting a photon in a large fraction of the possible time slots.
The single-photon problem
Putting all those features together in a single-photon source turns out to be a difficult challenge. Lasers emit a stimulated cascade of photons. A continuous-wave 1 µW beam from a 1 µm laser includes about 5000 photons per nanosecond. Spontaneous emission can produce one photon per atom, but light sources contain many atoms and spontaneous emission is not particularly orderly.
A fundamental issue is that the release of light energy is not deterministic at an atomic level. Each excited species has a characteristic lifetime, but that’s only a statistical measure of the probability that it will emit light, not a clock generating pulses at regular intervals. The probability of light emission during a given interval follows a Poisson distribution (see Fig. 1). To make sure a source emits no more than one photon during the chosen interval, the emission probability must be made so low that the source is most likely to emit no photons at all. As the chance of emitting one photon during an interval grows, so does the chance of emitting a second photon.
It is possible to simulate a single-photon source by strongly attenuating light pulses that initially contain many photons so only a single photon remains. That approach is widely used in quantum cryptography experiments, but is inherently very inefficient. Research is now focused on developing true single-photon sources that can do better.
Developers are targeting several key figures of merit. One is the efficiency, defined as the fraction of time slots occupied by single photons. A closely related figure is the probability the time slot will contain two or more photons instead of one. Another goal is to generate photons that are indistinguishable from each other. Additional goals include high repetition rates and a design that easily couples photons into a transmission fiber or free space. Current efforts are aimed at confining a light-emitting species-as a gas or solid-inside an optical cavity that helps control and couple emission.Trapped ions and atoms
Gas-phase single-photon sources isolate a single ion or atom within a resonant optical cavity (see Fig. 2). The atom or ion can be trapped, or can be one of a series falling through the cavity. An outside source excites it to emit a single photon resonant with the optical cavity. This design ensures that the same species always emits at the same wavelength, determined by the excited transition and the cavity.
Herbert Walther’s group at the Max Planck Institute for Quantum Optics in Garching, Germany, has generated single photons at 866 nm from calcium ions pumped optically with 397 nm pulses lasting up to 6 µs.1 Repeating at 100 kHz, they generated single photons in 4.6% of time slots-good for single-photon sources. Two photons were produced in only two of 400,000 single-photon events.Single atoms likewise can be excited to emit light resonant with an optical cavity. Jeff Kimble’s group at the California Institute of Technology in Pasadena has trapped single atoms long enough for them to emit a series of photons spaced more uniformly in time than is normal for lasers.2 The group’s single-atom lasers, however, do not emit single photons.
Gerhard Rempe’s lab at the Max Planck Institute for Quantum Optics is studying cooled rubidium atoms falling slowly through an optical cavity in vacuum. Early experiments generated a series of single-photon pulses on a stimulated Raman transition.3 Rempe is now trying to make a “photon pistol” that can fire single photons on demand.
Solid-state sources
The two leading solid-state single-photon sources are quantum dots and excitons bound to impurities. Both have an important advantage in speed. Current gas-phase systems generate pulses in the microsecond range, limiting their potential speeds to megahertz or less. Solid sources produce pulses of 10 to 100 ps, fast enough for pulse rates well into the gigahertz range.
So far, the more popular approach is quantum dots, which can be fabricated in various kinds of optical cavities. Typically the excitation source and/or the cavity design selects one quantum dot from among many to emit a single photon. Both optical and electronic excitation have been demonstrated. Each has its advantages. Optical excitation offers better performance and choice of transition levels. Electrical excitation promises devices that should be more practical to integrate into applications such as communications equipment.
Optical pumping was demonstrated first and has achieved good results. Some of the best have come from indium arsenide quantum dots sandwiched between distributed-Bragg-reflector mirrors. Etching away the surrounding material leaves tiny pillars, which confine light in three dimensions (see Fig. 3). This design improves efficiency and reduces the probability of two-photon emission. Andrew Shields’ group at Toshiba Research Europe (Cambridge, England) has measured reduction of two-photon emission by as much as a factor of 50.4
Yoshihisa Yamamoto’s group at Stanford University (Palo Alto, CA) demonstrated that micropillars have another key advantage-repeatedly exciting the same pillar-microcavity quantum dot made successive pulses nearly optically indistinguishable, yielding average overlap of successive pulses as large as 0.8.5 Quantum dots also can be made in photonic-crystal cavities.
Shields’ group at Toshiba was the first to demonstrate electrical pumping, by incorporating quantum dots into a p-i-n LED.6 Recently the group has extended the technique to the 1.3 µm telecommunications band.7
Quantum dots have significant limits as single-photon sources. Each dot has a unique wavelength and radiative lifetime, determined by its size and structure, so Yamamoto’s success in making indistinguishable photons required exciting the same single quantum dot each time.
Such limitations have led to interest in defect-based excitons, which all emit at identical wavelengths because their recombination lines are determined by the properties of the defect and the host semiconductor. That promises the ability to make an array of single-photon emitters with identical output, and more indistinguishable emitters, important for potential applications. However, gas atoms are ahead at the moment-in April a group led by Antoine Browaeys of the Laboratoire Charles Fabry de l’Institut d’Optique (Orsay, France) reported observing quantum interference between single photons emitted by rubidium atoms in two separate traps 6 µm apart.8
Outlook
“The field is evolving very rapidly,” says Yamamoto. But there are formidable challenges to overcome in developing practical single-photon emitters. The number of time slots filled by emitted photons is at best only a few percent. “We should improve the number to 50%,” says Yamamoto. Even with so few time slots filled, the probability of finding two photons in an occupied time slot is about 1%—too high to be acceptable. He also stresses the need to improve waveform overlap to virtually 100%, so photons can interfere with one another for quantum applications.
Yet, overcoming the barriers promises its own reward-realizing the potential of quantum information systems. New groups are entering the field, and advances continue to appear in such journals as Physical Review Letters, Nature, Optics Letters, and Optics Express. Keep watching for more developments.
REFERENCES
1. M. Keller et al., Nature 431,1075 (Oct. 28, 2004).
2. J. McKeever et al., Nature 415, 268 (Sept. 18 2003).
3. A. Kuhn et al., Physical Rev. Lett. 89, 067901 (Aug. 5, 2002).
4. A. J. Bennett et al., Optics Express 13, 50 (Jan. 10, 2005).
5. C. Santorl et al., Nature 419, 594 (Oct. 10, 2002).
6. Z.Yuan et al., Science 295, 102 (Jan. 4, 2002).
7. M. B. Ward et al., Applied Phys. Lett. 86, 201111 (May 16, 2005).
8. J. Beugnon et al., Nature 440, 779 (April 6, 2006).
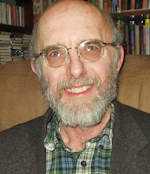
Jeff Hecht | Contributing Editor
Jeff Hecht is a regular contributing editor to Laser Focus World and has been covering the laser industry for 35 years. A prolific book author, Jeff's published works include “Understanding Fiber Optics,” “Understanding Lasers,” “The Laser Guidebook,” and “Beam Weapons: The Next Arms Race.” He also has written books on the histories of lasers and fiber optics, including “City of Light: The Story of Fiber Optics,” and “Beam: The Race to Make the Laser.” Find out more at jeffhecht.com.