NONLINEAR OPTICS: Slow light boosts electro-optic effect in lithium niobate
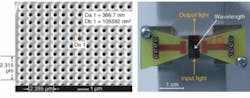
By adding a photonic-crystal (PC) structure to lithium niobate (LN) to slow light within the material, researchers at the Institute-Femto-ST (Besançon, France) drastically enhance the electro-optic effect, producing a miniature modulator that needs very little power to operate. The group velocity of the 1550 nm light is slowed by photonic band-edge effects; the device’s wavelength-shift sensitivity is 312 times that theoretically predicted for bulk material.
The PC pattern (a 15 × 15 square lattice of holes) is patterned on an annealed proton-exchange waveguide fabricated on a standard 500-µm-thick LN wafer, according to researcher Maria-Pilar Bernal. Electrodes on both sides of and parallel to the waveguide induce a refractive-index modulation via the Pockels effect (see figure).
The transmission spectrum was measured using a white-light source consisting of a mcrochip laser whose subnanosecond pulses at 1064 nm were coupled into 20 m of photonic-crystal fiber to produce a supercontinuum ranging from 600 to 1800 nm. Two stop bands were observed, one from 1125 to 1200 nm and the other from 1400 to 1550 nm. The edge of the latter band was used for intensity modulation. An applied voltage shifts the band edge by 2.5 nm/V; reversing the voltage reverses the direction of the shift, confirming the electro-optic effect. An applied voltage of 80 V changes the refractive index by 0.32.
“So far, we have experimentally measured a 3 dB bandwidth of 100 MHz,” says Bernal. “Our prototype has a very simple electrode design, which consists of two titanium strips of 150 nm thickness and 1 cm length. In addition, no impedance match was taken into account. With smaller electrodes on the order of 10 µm matched to 50 W and with an effective index matched with that of the PC structure, the LN PC modulators have the potential to reach the terahertz bandwidth. We are currently working on these improvements and results should come in the near future.”
Thin layers of LN
Benal notes that the biggest difficulty of working with LN is the fact that it is extremely difficult to create in thin layers to make a LN PC slab. “The main difficulty is keeping its crystalline and electro-optic properties,” she says. “Today it is difficult to envision a hybrid PC with materials like silicon or other semiconductors. However, this is certainly going to change in the next 36 months.” Bernal has initiated a European STREP (specific targeted research project) funded by the European Commission, “Single-step 3-D deposition of complex nanopatterned multifunctional-oxide thin films,” with the objective of growing thin layers of LN and certain other ferroelectric-family materials via laser chemical-beam epitaxy. “In my opinion, when we are able to produce photonic crystals in LN slabs, the devices will integrate well with any other type of PC device,” says Bernal.
In other applications, Bernal’s group is starting to develop LN photonic-crystal superprisms; along with other colleagues at Femto-ST, they are also working on what they call “phoXonic” crystals in lithium niobate. “These are structures that possess a simultaneous photonic and phononic bandgap,” she explains. “We believe that we can produce a new generation of acousto-optical devices.” In addition, doping the LN with erbium may result in interesting active devices, she says.
REFERENCE
1. M. Roussey et al., Applied Physics Lett. 89, 241110 (2006).
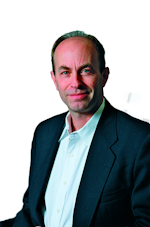
John Wallace | Senior Technical Editor (1998-2022)
John Wallace was with Laser Focus World for nearly 25 years, retiring in late June 2022. He obtained a bachelor's degree in mechanical engineering and physics at Rutgers University and a master's in optical engineering at the University of Rochester. Before becoming an editor, John worked as an engineer at RCA, Exxon, Eastman Kodak, and GCA Corporation.