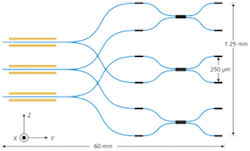
The search for exoplanets (planets orbiting other stars) has taken several forms; a well-known one is the transit method, in which the brightness of a star is monitored to spot any possible instances of a planet crossing in front of the star. Another form, and one with a special appeal to many people, is direct imaging. However, only a few very large and very hot (and thus bright in the IR) planets have been found using this approach.
But applying more-exotic imaging approaches may greatly boost the number of exoplanets that can be seen. Using nulling interferometry to cancel the light coming directly from a star, and interferometric imaging to create a synthetic aperture much larger than that of any existing optical telescope, it should be possible to find smaller and more-Earthlike exoplanets.
In a step toward practical stellar interferometric imaging, a group of scientists from the University of Michigan (U of M; Ann Arbor, MI) and the Laboratoire d’Astrophysique de Grenoble (Grenoble, France) have recently unveiled a very workable version of the central component in a stellar interferometer—the beam combiner.1 Designed to operate in the IR astronomical L-band between 3 and 4 µm (where exoplanets are expected to be bright in relation to their parent star), the device is a three-beam integrated-optic combiner fabricated in lithium niobate (LiNbO3), a material chosen for its transparency in the L-band.
Imitating a telescope
Interferometric imaging uses the light from two or more widely spaced mirrors to imitate the imaging ability of a telescope with an aperture equal to the maximum mirror spacing. All interferometers have beam combiners; however, to create a true 2-D image (as opposed to imaging along only one dimension), the beam combiner must accept more than two beams, splitting each beam and then combining the result in various two-beam combinations.
By far the most rugged and stable way to do this is with integrated optics; however, in the valuable K-band, the normally used silicate-based glasses are not transparent. To create an integrated-optic beam combiner that transmits in the K-band and also allows complex configurations with more than two beams, the U of M and Grenoble researchers chose titanium-indiffused X-cut LiNbO3—a material that also allows electro-optic modulation.
They created a three-beam combiner on a substrate 60 mm long by 20 mm wide, with a 1 mm thickness; the integrated-optic portion resides in a 1.25-mm-wide strip along the center of the substrate’s surface (see Fig. 1). The waveguides are all single-mode, and thus spatially filter their inputs. The combiners themselves are all 3 dB symmetric directional couplers. Electro-optic phase modulation (which is essential in taking the many measurements that are then assembled to synthesize the final image) is accomplished via thin-film metal electrodes. The researchers also fabricated a two-beam combiner for laboratory testing.The small refractive-index difference (0.0136) between the waveguide and the surrounding material creates potentially large bending losses, so the radius of curvature for the S bends in the optical circuit was chosen to be 22 cm, which limits losses to 0.64 dB/cm at 3.39 µm for the TE mode and 2 dB/cm for the TM mode. Crosstalk at the X-shaped waveguide crossing was measured to be below 20 dB.
White-light fringes
A laboratory experiment was set up to generate white-light (broadband) fringes with the two-beam combiner using a blackbody (1170 K) thermal source. The output from the combiner’s directional coupler was imaged onto a cooled indium antimonide detector, with a lock-in amplifier used to make the measurement. The measured fringe closely matched the theoretical fringe (see Fig. 2). Future devices will require the use of techniques to suppress this wavelength dependence, say the researchers—especially for stellar-interferometric setups that will be based on precise nulling to cancel starlight to make exoplanets as noticeable as possible.
REFERENCE
- Hsien-kai Hsiao et al., Optics Express 17(21) p. 18489 (Oct. 12, 2009).
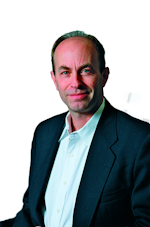
John Wallace | Senior Technical Editor (1998-2022)
John Wallace was with Laser Focus World for nearly 25 years, retiring in late June 2022. He obtained a bachelor's degree in mechanical engineering and physics at Rutgers University and a master's in optical engineering at the University of Rochester. Before becoming an editor, John worked as an engineer at RCA, Exxon, Eastman Kodak, and GCA Corporation.