PHOTONIC FRONTIERS: LASER REMOTE SENSING: New needs and technology push laserremote sensing in the atmosphere
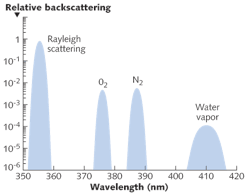
New user requirements and new technology are revitalizing the venerable field of laser remote sensing of the atmosphere. Lidar (for LIght Detection And Ranging) systems have been collecting atmospheric data from the ground, air, and space for four decades. That data is important input for computer weather and climate models, as well as for atmospheric research. Sometimes laser instruments provide key information for unexpected needs, such as tracking the spread of volcanic ash from Iceland that grounded European air traffic in April.
Atmospheric sensing has benefited greatly from continuing improvements in lasers, detectors, and signal-processing technology. Thirty years ago, a lidar based on a lamp-pumped Q-switched solid-state laser would have cost $100,000 to $200,000, says Dennis Killinger of the University of South Florida (Tampa, FL). Diode-pumped solid-state lasers improved efficiency and performance. Now, lidars based on pseudo-noise-modulated continuous wave or high pulse-repetition-frequency nanosecond pulsed fiber lasers can cost as little as $10,000 to $20,000, and their higher efficiency reduces power and cooling requirements. Those developments are making lidars more affordable as well as suitable for a wider range of environments, and a federal interagency panel is developing a proposal to build 400 new lidar-equipped atmospheric measurement stations spread across the United States to collect data for weather forecasting and climate research.
Types of atmospheric lidars
The simplest type of atmospheric lidar systems are ceilometers, ground-based instruments that measure the altitude of cloud bases by firing nanosecond pulses from a diode laser straight up and timing their return. Clouds or precipitation stand out because they scatter light more strongly than the background air.
Other lidars measure Rayleigh or Raman scattering within a body of transparent air. Their range depends on the laser power and signal-processing system.
Rayleigh scattering is at the same wavelength as the transmitted laser pulse, which makes detection more complex than in simple laser radars because the scattering comes from a large volume of air, not from a single discrete object. The return signal contains information on two unknown quantities—the backscatter coefficient and the atmospheric transmission—so in practice one of the unknowns is assumed (based on a model or other information) so the other can be calculated from the measured data. Such lidars are relatively simple and are valuable for profiling the distribution of light-scattering aerosols.
Raman scattering is inelastic, so light is scattered at a wavelength shifted from the laser wavelength by an amount characteristic of the scattering molecule. This has the advantage of shifting wavelengths enough to reduce noise levels, but the disadvantage of being typically four orders of magnitude less efficient than the elastic return at the laser wavelength, and thus requiring high-power pulses to yield adequate return signals. Because each molecule has a characteristic Raman shift, observing a range of Raman-scattered wavelengths can identify multiple species, and known concentrations of common gases such as nitrogen can be used for a precise measurement of the atmospheric pressure (see Fig. 1). Simultaneous measurements of Raman scattering from nitrogen and water vapor gives the mixing ratio of the two species (see Fig. 2).Each component of the atmosphere has its own characteristic Raman shift, so detecting a range of wavelengths can measure multiple species. The most common approach is to simultaneously measure water vapor and nitrogen scattering, with the nitrogen data divided into the water vapor scattering to give a mixing ratio. A dual detection system, which measures both Rayleigh scattering from aerosols and Raman scattering from nitrogen, can measure the mixing ratio of aerosols to nitrogen, without the assumptions needed when only observing Rayleigh scattering.
Doppler lidars—like Doppler radars—measure a shift in the frequency of the returned signal, so they can measure wind velocity. They are particularly valuable for measuring small-scale effects such as wind shear and turbulence. Several airports prone to such effects have deployed Doppler lidar systems, says Mike Hardesty, chief of the atmospheric lidar division at the National Oceanic and Atmospheric Administration (Boulder, CO). They also can profile airflow around wind turbines and potential wind-power sites, both to assess potential power yield and to study turbulence and wind shear that might degrade turbines.
Differential absorption lidars profile levels of trace components of the atmosphere by emitting paired pulses at two closely spaced wavelengths, one at the peak of an absorption line, the other off-peak. The difference in return signals measures the concentration of gas such as nitrogen dioxide, ozone, and water vapor.
Many of these systems are used on the ground for observations of overhead conditions, but others are deployed in satellites or flown for look-down measurements.
Lidar technology
Solid-state lasers are standard for all lidars except for ceilometers. High peak power in a short pulse gives the best signal-to-noise ratio in return measurements. Early lamp-pumped Q-switched lasers could observe beyond 5 km. By the 1990s, diode-pumped Q-switched solid-state lasers producing nanosecond pulses could observe at 5 to 10 km, opening new opportunities. James Ryan of the University of New Hampshire had initially considered using a lamp-pumped laser in a balloon-borne lidar system, but its low efficiency and cooling requirements made the system impractical. He notes, "We thought we were done for," until he switched to a diode-pumped laser. Many new lidars use fiber lasers that produce trains of picosecond pulses, together with photon counting systems based on avalanche photodiodes to pick up weak signals, which can make measurements out to several kilometers.
Another trend has been toward the use of eye-safe wavelengths, which allow the use of higher powers in areas where people or aircraft might be exposed to the beam. Erbium-doped fiber lasers typically operate at 1.55 μm, and holmium-thulium-YLF lasers at 2.0 µm can measure atmospheric carbon dioxide. The development of photon counting detectors for wavelengths as long as 2.5 to 3 µm has improved performance.
Atmospheric measurement requirements
The new technology comes at an opportune time, when the U.S. needs to overhaul its aging ground-based systems for measuring vital weather and climate data. Existing networks that collect data on the scales needed for weather forecasting and fine-resolution climate models are "highly variable in quantity, quality, accessibility, instrument set, site selection, and metadata," wrote a National Research Council panel in a 2009 report titled "Observing Weather and Climate from the Ground Up: A Nationwide Network of Networks."1 The panel added, "The ability to measure atmospheric conditions at various heights ... is particularly inadequate."
Computer models that forecast weather and study climate differ in many details, such as time scale, geographic resolution, and extent, and how fast they must run to yield useful results. But they share a need for consistent measurements at regularly spaced locations of key atmospheric parameters including wind speeds, humidity, aerosols, air quality, and temperature. This data goes into a three-dimensional grid, which the models use to forecast trends.
Lidars perform three of the four observations the report lists as most in need of improvement and critical for predicting "high-impact" weather:
- Height of the planetary boundary layer, separating surface air circulation from higher-altitude winds
- High-resolution vertical profiles of humidity
- Measurements of air quality and related chemical composition above the surface layer.
To meet these needs and improve other data collection, the panel urged installing lidar systems along with radio-frequency wind profilers at some 400 new stations spaced at an average of 125 km across the U.S. to collect wind and humidity profiles and observe the boundary layer. Lidar details are to be determined, but the goal is to collect a uniform and comprehensive data set.
This would be the first large-scale deployment of lidars. The challenge, says Hardesty, is to develop laser systems that can be made cheaply enough to be deployed in such quantity but are powerful and robust enough to probe the atmosphere several kilometers away.
Measuring volcanic ash
Lidars hit the news in April, when they were used to monitor fine volcanic ash blasted high into the atmosphere by an Icelandic volcano. The dust that grounded aircraft across much of Europe was invisible to people looking up, but lidar observations revealed it clearly. Four British lidar stations operated by the University of Manchester measured ash layers a few hundred meters thick slowly descending toward the boundary layer for four to five days. That data was very important for the British Meteorology Office because "they had no other way to validate their dispersion model," says Geraint Vaughan of Manchester.As soon as it heard of the eruption, MeteoSwiss switched the 355 nm lidar built by the Swiss Federal Institute of Technology (EPFL) that normally monitors relative humidity to collecting aerosol data, says Bertrand Calpini, head of the MeteoSwiss Aerological Station Payerne (Payerne, Switzerland). The lidar extracted the aerosol backscattering ratio from the ratio of aerosol Mie elastic scattering and rotational Raman scattering from N2 and O2, yielding the only ash-cloud elevation data available to Swiss air space managers. But Calpini said they really needed absolute concentration of the ash, a result that took him and colleagues at EPFL three days to produce because they needed additional data on the ash collected by other instruments to calibrate the lidar.
Outlook
With another Icelandic volcano rumbling uneasily, European planners are looking at ways to better use their lidars to monitor future ash clouds.
The NRC report has stimulated "a bevy of activity in Washington," says Richard Carbone of the National Center for Atmospheric Research (Boulder, CO), who chaired the panel. Some 22 federal agencies are working fiscal 2012 budget proposals, coordinated by the Office of the Federal Coordinator of Meteorology. Major agencies such as NASA, NOAA, and the Department of Defense are enthusiastic, he adds. Separately, the American Meteorological Society has a parallel effort involving private companies plus hundreds of state agencies concerned with water, air quality, emergency management, and other related issues.
That's encouraging news. After four decades of development, it's about time to put laser atmospheric sensing to work collecting the data we need to improve weather forecasting and our understanding of climate.
REFERENCE
- "Observing Weather and Climate from the Ground Up: A Nationwide Network of Networks," National Academies Press 2009, http://www.nap.edu/catalog.php?record_id=12540.
About the Author
Jeff Hecht
Contributing Editor
Jeff Hecht is a regular contributing editor to Laser Focus World and has been covering the laser industry for 35 years. A prolific book author, Jeff's published works include “Understanding Fiber Optics,” “Understanding Lasers,” “The Laser Guidebook,” and “Beam Weapons: The Next Arms Race.” He also has written books on the histories of lasers and fiber optics, including “City of Light: The Story of Fiber Optics,” and “Beam: The Race to Make the Laser.” Find out more at jeffhecht.com.