PHOTONICS APPLIED: DEFENSE: IR countermeasures aim for safer flights
An infrared countermeasure—a device designed to prevent a heat- or plume-seeking missile from reaching its target—generally consists of a flare, laser, or other bright illumination source and optics combination that, when placed on an aircraft or marine craft, confuses a missile’s target acquisition system.
Today, infrared countermeasure (IRCM) systems are standard equipment for most military aircraft. But although the US House and Senate introduced bills H.R. 2780 and S.1193 in 2005, each called the Commercial Airline Missile Defense Act, neither became law. Nonetheless, progress is being made in the commercial airlines arena: More powerful lasers and improved optoelectronic and optomechanical components are reducing the size and weight of IRCM systems, bringing them a step closer to accompanying you on your next flight home.
IRCM technology
While much of the technology in modern IRCM is proprietary, each system basically consists of a missile-tracking system with IR cameras and optics, the countermeasure light source or “jammer” that confuses an approaching missile (the “seeker”), a data-processing system for pattern recognition and control functions, and mechanical components that may translate/rotate the imager and light source.
“All seekers target a jet-engine plume in a somewhat narrow field of view and modulate the detected signal in order to track the location of the source energy in relation to the missile’s flight direction,” says William Caplan, engineering consultant at Netherlands Infrared Consulting and Modelling (NIRCM; The Hague, the Netherlands). “The job of the jammer is to provide a higher-intensity modulated source in the same field of view as the aircraft heat source being targeted [see Fig. 1]. In the case of directional IRCM [DIRCM], the source is mounted on a turret that directs the laser beam to the seeker. The jammer signal confuses the missile-tracking electronics, breaking their ability to lock onto the original IR source.” Caplan says that different seekers operate with different spectral bands, say 1–3 µm or 3–5 µm, for example, meaning that IRCM must broadcast a jamming signal in all bands. “MANPADS [man-portable air defense systems] technology hasn’t changed much in 20 years, and seekers typically target shortwave- to midwave-infrared [SWIR to MWIR] signals. Fortunately, imaging and visible-band seekers are not widely available to illicit [terrorist] groups,” adds Caplan. “Nonetheless, IRCM manufacturers need to anticipate probable increases in the sophistication of enemy combatant technology and design jammers with multispectral, multiband emissions that span the seeker detection bands. Considering that MANPADS can acquire and intercept a target in 2–10 s, the jammer needs to be effective and fast.”
BAE Systems’ (Nashua, NH) IRCM has been used during Desert Storm, Bosnia, Kosovo, and numerous other operations. The company’s ALQ-144A onboard jammer with offboard flares protects a target against old and new IR threats, while its AN/ALQ-212(V) advanced threat IR countermeasures/common missile warning system (ATIRCM/CMWS) suite provides protection against all IR threat bands, successfully defeating 10 of 10 IR signature analysis models in live-fire tests. BAE’s IRCM system detects an approaching missile, rejects any false alarms, and cues the onboard IR jamming system’s jam head to the missile’s location. When the jam head finds the missile with its IR tracking system, it emits a high-energy IR beam to defeat the missile’s IR seeker and incorporates one or more IR jam heads to counter multiple missile attacks.
Northrop Grumman (Rolling Meadows, IL), who built the first IR jamming laser, now has 2000 Viper lasers in service since 2002. Viper is a diode-pumped Nd:YAG laser that uses periodically poled lithium niobate (PPLN) to perform wavelength conversion in a 13 × 2 in. housing weighing less than 10 lb. Northrop Grumman calls Viper a true multiband laser, emphasizes the importance of co-alignment of the different-wavelength lasers, but like all IRCM manufacturers, cannot comment on the precise laser specifics or modulation schemes.
“We can say that lasers have made the optical break lock possible, whereby a missile violently and instantaneously turns away from its target upon seeing the laser signal,” says Jack Pledger, Northrop Grumman director of IRCM business development. “The jamming intensity to target heat-signature intensity ratio, or J/S, has grown from 10/1 for lamp-based DIRCM to nearly 1000/1 for laser-based DIRCM, upping the requirements for IRCM lasers in order to keep pace with missile detection advances. Thulium-fiber lasers and QCLs [quantum-cascade lasers] show great promise in reducing cost, weight, and increasing reliability over the -45º to +40ºC IRCM operating environment,” adds Pledger.
Multiple manufacturers are rising to the mid-IR laser challenge. “Our QCL-based JammIR laser system differs from Northrop Grumman’s Viper OPO [optical parametric oscillator], BAE Systems’ multiband laser OPO, and Elbit’s MUSIC fiber-laser pumped systems in a number of ways,” says Eric Takeuchi, director of business development for Daylight Defense (San Diego, CA). “Using QCLs in a modular architecture lets system integrators pick and choose wavelengths and power levels that suit their requirements.” Takeuchi says that QCL systems have a much smaller parts count for improved reliability and operate directly from injected current, meaning electrons in and photons out at the correct wavelengths. “Conversely, Viper OPO and thulium fiber-laser-pumped OPOs require a series of optical pumping and nonlinear frequency conversion steps in order to generate the correct wavelengths for defeating heat-seeking missiles, resulting in very complex laser systems with significant cost and reliability disadvantages.” The 9.5 lb JammIR “Series B” QCL systems, adds Takeuchi, operate over an extremely wide temperature range from -54º to +71ºC, have been flight-tested on a wide variety of military helicopters, have been demonstrated to defeat heat-seeking missiles, and operate with only solid-state thermal management—no evaporative cooling required.
ITT (Clifton, NJ) is relying on Daylight Defense’s QCL technology for the Army’s upcoming Common Infrared Countermeasures (CIRCM) competition. The program will bring IRCM capability to the Army’s 4000-plus light-to-medium weight helicopters and is valued between $1.5 and $2 billion over the next five years. “ITT was one of the first defense companies to recognize the potential of QCL technology for military IRCM processes, and we’ve been working with Daylight to get this particular laser up to military requirements,” said Bob Lawler, ITT Electronic Systems’ director of Army Programs. “QCLs offer more power and higher reliably at a lower weight than existing lasers and, for our purposes, all three of those elements are critical to a successful system,” added Lawler.
Wall-plug efficiency (WPE) is another key attribute of mid-IR QCLs. Pranalytica (Santa Monica, CA) uses its nonresonant extraction (NRE) QCL designs to achieve WPE values of 10% or better over wavelengths covering the 3.6–17 µm spectral range, in both cooled and uncooled options. Continuous-wave, room-temperature MWIR output power levels are typically >2 W at the short-wavelength end and >4 W at the long-wavelength end of interest. Pranalytica also has a multiple-wavelength system with multiwatt power output for two or more wavelengths in the MWIR region and a 2.0 µm window in the SWIR region with a collimated, collinear output beam; all modules meet MIL-STD 810G vibration/shock and temperature requirements.
Commercial obstacles and opportunities
“There are three main pillars of resistance to the deployment of IRCM on commercial aircraft: cost, weight, and drag,” says NIRCM’s Caplan. “With a complete IRCM system estimated to cost around $1 or $2 million, you can already see the limitations to widespread adoption of the technology.1 Another figure debated in the industry is a 3% increase in fuel consumption due to system weight and increased aerodynamic drag for IRCM-equipped aircraft, which is huge for commercial airlines.”
Caplan, who worked on DIRCM implementation as a principal scientist at the North Atlantic Treaty Organization (NATO; www.nato.int) for more than a decade, sees possibility for progress in overcoming these obstacles, however. “After [9/11], the attacks on a commercial airliner in Mombasa, Kenya in 2002 and a DHL aircraft in 2003 tremendously raised awareness of the MANPAD threat to civil aviation.” In fact, a 2006 report in Aerospace America estimated that there have been 35 MANPADS attacks on civil and commercial aircraft, resulting in as many as 500 fatalities. The report also estimated that US IRCM funding was forecast to grow from just under $600 million in 2006 to nearly $1 billion in 2009 and beyond, with BAE Systems and Northrop Grumman each holding a roughly 40% IRCM market share in 2006.2Indeed, Northrop Grumman is celebrating a milestone this year: the 50th anniversary of its development of the first IRCM system.3 “In response to DHS [US Department of Homeland Security] counter-MANPADS requirements, we have taken the components of a military IRCM—cameras, lasers, optics, electronics—and put them in our removable 500 lb Guardian pod that can be mounted to a commercial aircraft in 10 minutes with two people,” says Pledger (see Fig. 2). “Our commercial airline partners prefer a podded approach so that IRCM can be installed only for active aircraft flying in high-threat geographical locations or during threat-elevated situations. We have 20,000 hours of flight time with the pods on FedEx airplanes and have shown that the increased fuel requirement is not even calculable.” As for the high cost of the systems, Pledger says, “Cost is a red herring. The best way to reduce cost below $1 million is through economies of scale. We’ve estimated that a $1 per passenger surcharge would pay for a reasonable number of IRCM pods to protect a fleet in high-threat locations and situations for 20 years of service.”
But Northrop Grumman is not the only name in commercial IRCM. At the Paris Air Show 2011 (www.paris-air-show.com), Elbit Systems Electro-Optics, or Elop Ltd. (Rehovot, Israel), displayed its commercial DIRCM pod; in fact, Elop is the recipient of the first contract to provide DIRCM systems to commercial airlines.4 “After 10 years of analyzing the operation needs of commercial airlines and drawbacks of current DIRCM systems, we leapfrogged the competition on both the laser level and the gimbal technology,” says Dan Slasky, vice president of electro-optic systems and lasers for Elop. “Our Multi Spectral Infrared Countermeasure system—called MUSIC—uses fiber laser technology in a very small housing and gains such inherent advantages as high reliability and lower cost, size, and weight with higher efficiency across a broad spectral range, while our gimbal sheds antiquated external mechanics and is contained inside a closed, sealed dome—no moving parts outside the aircraft.” Slasky says Elop’s 2.7-m-long commercial MUSIC (C-MUSIC) DIRCM pod is half the height and weighs less than competitive systems, reducing aircraft drag to extremely low levels. And the rapid gimbal action and very wide field of view of the cameras that comprise the C-MUSIC missile-warning system make it ideal for large aircraft such as Boeing 747 and Airbus 320 class airplanes. Elop, says Slasky, has been extremely successful at marketing MUSIC and C-MUSIC not only to military and civilian airlines, but to cargo companies, companies that fly contractors into sensitive military zones, and for VIP aircraft companies.
Considering the swarm of civil uprisings in recent months, here’s hoping that governments and private institutions find a way to make commercial IRCM a reality. After all, says Caplan, “I’d like to think that our industry or regulators won’t wait for a disastrous terror attack—that could have been prevented—before implementing lifesaving IRCM technology.”
REFERENCES
1. D.L. Rockwell, Aerospace America (May 2002); http://bit.ly/mXVgTJ.
2. D.L. Rockwell, Aerospace America (April 2006); http://bit.ly/pgVZXW.
3. Northrop Grumman company announcement (June 21, 2011); http://bit.ly/kHUPh2.
4. Elbit Systems company announcement (June 25, 2009); http://bit.ly/r7AXbQ.
Coming next month in the “Photonics Applied” series...
Not all solar simulators are created equal
A solar simulator makes possible accurate measurement of the optical-to-electrical conversion efficiency of solar photovoltaic (PV) cells. The instrument quantitatively simulates the irradiance and spectral output of our own Sun so that PV manufacturers can obtain efficiency data without leaving panels to bake in unpredictable outdoor environments. Xenon-light-source-based simulators are available from numerous companies and are distinguished by factors like whether they are Class A, B, or C, steady state or pulsed, and how well they match the solar spectrum and maintain uniformity over time. In this article, senior editor Gail Overton examines the cost and performance of available simulator technologies and the related tradeoffs.
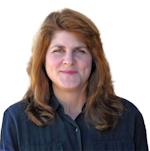
Gail Overton | Senior Editor (2004-2020)
Gail has more than 30 years of engineering, marketing, product management, and editorial experience in the photonics and optical communications industry. Before joining the staff at Laser Focus World in 2004, she held many product management and product marketing roles in the fiber-optics industry, most notably at Hughes (El Segundo, CA), GTE Labs (Waltham, MA), Corning (Corning, NY), Photon Kinetics (Beaverton, OR), and Newport Corporation (Irvine, CA). During her marketing career, Gail published articles in WDM Solutions and Sensors magazine and traveled internationally to conduct product and sales training. Gail received her BS degree in physics, with an emphasis in optics, from San Diego State University in San Diego, CA in May 1986.