TERAHERTZ INSTRUMENTATION: Terahertz technology enables systems for molecular characterization
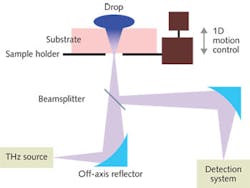
In addition to its nonionizing nature and its ability to penetrate nonmetallic objects, terahertz radiation is sensitive to the motions (vibrational, rotational, torsional, and translational) of molecules, allowing high-sensitivity spectral probing of molecular events in areas of practical importance. Applied Research & Photonics has leveraged these attributes to utilize broadband (approximately 30 THz), high-power (>5 mW) continuous-wave (CW) terahertz radiation generated from an electro-optic dendrimer that enables smart spectrometer and scanning reflectometer systems for molecular characterization.
Electro-optic terahertz generation
The electro-optic (EO) method of terahertz generation is advantageous because the pump-terahertz conversion is not limited either by emission saturation or heat dissipation. The EO route main mechanisms include EO rectification (EOR) and difference frequency generation (DFG). Of these, EOR depends on the introduction of an ultrafast (femtosecond) laser pulse into the lattice of an electro-optically active material; the lattice acts as a rectifier to convert the very high frequency derived from the ultrafast laser pump to a relatively lower frequency pulse that falls in the terahertz range—the so-called EOR effect.
The EOR method usually uses an 800 nm pulsed femtosecond laser, although other wavelengths such as 1064 nm may be used. The difficulty here is that two vital parameters—output power and the terahertz range—are completely dependent on the characteristics of the femtosecond laser. As a result, only low average power and a range of several terahertz (up to 5 THz) has been possible, making it difficult to uniquely characterize many materials systems.
In contrast, the DFG techniques not only eliminates the use of an expensive femtosecond laser, but can also produce both CW and pulsed terahertz radiation, as well as higher output power and broad-range tunability. Nobel Laureate Robert F. Curl, Jr. and colleagues reported generation of tunable far-infrared (IR) radiation by means of two singlemode laser diodes by focusing the overlapped beam in silver gallium sulfide (AgGaS2) crystal.1 Our earlier work showed that chromophore-doped and -poled poly(amido amine) dendrimer can produce approximately 3.4 mW of terahertz power (PTHz) when pumped by two fiber-coupled laser diodes with a combined pump power of around 5.5 W (Ppump).2 Thus the terahertz figure of merit [PTHz/(Ppump)2] of this source is 1.124 × 10-4 W-1. This value was achieved by means of the higher EO coefficient of the EO dendrimer: 130 pm/V.
Using DFG, the resulting terahertz frequencies are given by the difference of the pump laser frequencies, or νTHz is proportional to ν1 - ν2, meaning one can choose appropriate pump frequencies to obtain the desired output terahertz bandwidth. In practice, however, both ν1 and ν2 are not single-frequency lasers because laser diodes always have a bandwidth distribution around their main peaks; that is, when a stationary beam of the generated terahertz radiation is scanned by a moving beam derived from the same source, a wide frequency distribution will result. This mechanism is a variation of two-photon excitation.3
The terahertz scanning reflectometer
Measurement of the concentration gradient of a biological or other fluid in a noninvasive (and nondestructive) fashion is important in several areas, including penetration of an active ingredient through human skin or other tissues. But to our knowledge, there was no direct method—until now—to obtain two critical factors in such studies: 1) the concentration gradient of the permeating ingredient across the thickness of a substrate and 2) the kinetics (or rate) of such permeation. These two factors are essential for quantitative analysis, for example, via Fick’s laws of diffusion.4
In one dimension, Fick’s first law relates the flux, J, directly to the concentration gradient via J = -D(∂C/∂x), where C is the concentration and D is the diffusion coefficient. Fick’s second law relates the kinetics of diffusion with the second derivative of concentration gradient or ∂C/∂t = D(∂2C/∂x2). Therefore, direct measurements of the quantities ∂C/∂x and ∂C/∂t are possible. Our terahertz scanning reflectometer (TSR) is therefore capable of measuring both the concentration gradient and the kinetics of diffusion in real time, enabling life science and physical science advances in cell characterization, transdermal drug delivery, personal care products, and substrate/active ingredient characterizations where the effect of an active substance on a substrate is important.
For our TSR, CW terahertz radiation is generated from an electro-optic dendrimer via the DFG method (see Fig. 1). The terahertz beam is focused onto the specimen at a 90° angle using an off-axis parabolic reflector (normal incidence). The beam reflected by the substrate is directed to the detection system using a beamsplitter/combiner and the specimen cell is comprised of a scanning platform that is controlled by a one-dimension motion controller.
To make a direct measurement, the off-axis parabolic reflector is adjusted such that (initially) the terahertz beam remains focused on the substrate surface. At this position, the motion controller is engaged to scan the substrate and interrogate the reflectance across its thickness; this gives the ∂C/∂x parameter when the blank substrate reflectance is subtracted from the reflectance of the same substrate treated with a desired ingredient. However, when the beam remains focused at the surface and the motion control is locked at that position, then the ingredient is applied on the substrate to let it permeate across the thickness while the reflectance is measured in real time. In this case the reflectance is directly proportional to the rate of permeation of the ingredient across the substrate, or ∂C/∂t.
Terahertz scanning reflectometer measurements of the stratum corneum—the outermost layer of the skin’s epidermis—were characterized to obtain the permeation parameters for N-0915, a chemical known as a permeation retarder (see Fig. 2). First, the reflectance is measured for a blank stratum corneum and then, for the stratum corneum treated with N-0915. The difference of the two profiles determines the gradient ∂C/∂x and thus is a direct measurement of the concentration profile.We also measured the diffusion kinetics of water into photographic paper. The paper was mounted on the reflectometer and 25 µL of deionized water were dispensed (see Fig. 3). Here, the kinetics of penetration was measured in real time. The time-dependent change of reflectance is assumed to be a direct measure of the penetration kinetics, thus quantifying ∂C/∂t. Knowing both ∂C/∂t and ∂C/∂x, the diffusion coefficient can be calculated directly from Fick’s second law. All measurements are controlled from the front-end user interface with a Windows-based personal computer.
Terahertz spectroscopy
In common techniques such as Raman or IR spectroscopy, a sample is illuminated with a laser beam and the light is collected by a lens and passed through a monochromator. Wavelengths close to the laser line, due to elastic Rayleigh scattering, are filtered out while the rest of the collected light is dispersed onto a detector.
Modern Raman instruments use notch or edge filters for laser rejection and spectrographs—either axial transmissive (AT), Czerny-Turner (CT) monochromator, or Fourier transform (FT) spectroscopy based—and CCD detectors. But because spontaneous Raman scattering is typically very weak, it is difficult to resolve the weak, inelastically scattered light from the intense Rayleigh-scattered laser light. This fundamental limitation of Raman spectroscopy makes it difficult to resolve many molecules, especially those with closely spaced spectral lines.
When terahertz radiation interacts with molecules, it may stimulate many resonances such as molecular vibrations, phonons, and/or other resonances in the system, affecting the terahertz photons by characteristic amounts based on a specific interaction or event. The change in energy and/or frequency yields information about the molecular nature of the interaction. While IR and Raman spectroscopy yield similar information, they cannot detect as many resonant states as can terahertz spectrometers because terahertz photons are sensitive to the vibrational states of the entire molecule as opposed to just a bond or charge state.
Molecular simulation, especially molecular dynamics, reveals the numerous vibrational and conformational states possible when a molecule is not at its lowest energy state. Because most material remains at its lowest energy state under normal and steady state conditions, terahertz perturbation will stimulate possible available states in these regions. For example, terahertz spectroscopy can be used to analyze the single nucleotide polymorphism (SNP) of two single-stranded DNA molecules; SNP is a DNA sequence variation that occurs when a single nucleotide (A, T, C, or G) in the genome differs between members of a biological species or paired chromosomes in an individual (see Fig. 4).The detection of SNP has major implications in diagnostics and personalized medicine: It has been discovered that if one’s gene has one kind of SNP then certain drugs will be effective, whereas the lack of that SNP type means that the same drug will not work. If a doctor is able to easily identify the presence or absence of a given SNP in one’s gene, then they can prescribe the right drug.
Scientific studies reveal that DNA always goes through a process of switching between single- and double-stranded modes to create new proteins or to interact with enzymes/other proteins. The terahertz spectrometer is so sensitive, it can discern between single-stranded DNA and double-stranded (hybridized) DNA, making the instrument crucial to a number of biological studies.
REFERENCES
1. U. Simon et al., Opt. Lett., 18, 13, 1062 (1993).
2. A. Rahman, “Stimulated emission of terahertz radiation from electro-optic Dendrimer,” Proc. SPIE 7601: Terahertz Technol. and Applications III, San Francisco, CA, 76010C (Feb. 18, 2010).
3. A. Rahman, “Dendrimer Based Terahertz Time-Domain Spectroscopy and Applications in Molecular Characterization,” J. Molecular Structure, 1006, 59–65 (2011).
4. E. De la Barrera, Nat. Structural & Molecular Bio., 12, 280 (2005).
Anis Rahman | CTO and President, Applied Research & Photonics (ARP)
Anis Rahman is president and chief technology officer at Applied Research & Photonics (ARP; Harrisburg, PA).
Aunik K. Rahman | Senior Engineer, Applied Research & Photonics (ARP)
Aunik K. Rahman is a senior engineer at Applied Research & Photonics (ARP; Harrisburg, PA).