PHOTONICS APPLIED: LIDAR: Wind energy gets a boost from wind-turbine lidar
Light detection and ranging (lidar) technology has numerous applications in atmospheric sensing, topographical and agricultural assessments, and for three-dimensional (3D) industrial mapping and spatial analysis. But one of the most exciting applications for lidar is its use in estimating wind speed and direction to improve the performance of modern-day wind turbines that play an important role in the renewable energy future of our planet.
While many commercial wind farms operate without wind-turbine lidar, the scientific community is producing smaller, cheaper, more robust systems that are slowly gaining traction—and boosting power output—in the wind energy sector.
Boosting energy
According to the World Wind Energy Association (www.wwindea.org; Bonn, Germany), the world market for wind turbines continues to grow exponentially and reached a total size of 42 GW in 2011 (an all-time high), bringing the total wind energy capacity close to 239 GW—or 3% of the worldwide electricity demand (see Fig. 1).
In addition to the use of ground-mounted lidar stations to predict the best locations for a wind farm based on wind-assessment data, laser-based anemometers or wind-turbine lidar systems mounted on the nacelle, spinner, or blades of a wind turbine boost the turbine's energy production by anticipating the wind speed and direction ahead of the turbine blades and optimizing the blade parameters. While this energy increase is typically less than 5%, some companies claim improvements of 10% or more.1
Today, commercial wind-turbine Doppler lidar systems include continuous-wave systems such as ZephIR from Natural Power (Castle Douglas, Scotland) and pulsed-laser designs including Wind Iris from Avent Lidar Technology (Paris, France) and the Vindicator Optical Control System from Catch the Wind (Chantilly, VA).
Continuous-wave lidar
"Although lidar applications to wind energy were explored in the 1980s, the systems at that time were too large and expensive for serious acceptance," says Alex Woodward, product development and marketing manager at Natural Power, a renewable energy (wind-wave-tidal-biomass) consultancy and products company. "But the situation has changed dramatically, with rapid growth of the wind industry coinciding with a new generation of lidar based on optical fiber and other components that emerged from the telecommunications boom of the 1990s. The first all-fiber lidar systems were demonstrated in the late 1990s, and a commercial prototype unit called ZephIR was mounted on a turbine to demonstrate wind speed detection in front of the rotor plane in early 2003."
Since 2003, nearly 600 lidar deployments in the ZephIR family have been commissioned, including multiple, often confidential installations in commercial wind-turbine applications. Originally developed by QinetiQ (Malvern, England), ZephIR is exclusively offered by Natural Power/Zephir Ltd. in the UK, Europe, Canada, and Asia-Pacific areas and by Campbell Scientific (Logan, UT) in the USA.
Operating at laser Class 1 in the eye-safe band around 1550 nm, ZephIR continuous-wave (CW) coherent Doppler lidar systems illuminate a target—in this case particles in the air ahead of a wind turbine—at approximately 1 W average power levels and a small fraction of the light is backscattered into a receiver. Motion of the target along the beam direction leads to a change in the light's frequency via the Doppler shift—a frequency increase for motion toward the lidar system (a "blue shift"), and reduced frequency (a "red shift") as movement away stretches the wave. This frequency shift is measured by mixing the return signal with a portion of the original beam—the reference beam—and sensing the resulting beats at the difference frequency on a photodetector.
The reference beam, or local oscillator (LO), plays a crucial role in the measurement process. First, it defines the region of space from which light must be scattered for detection of the beat signal; radiation from other sources (like sunlight) is rejected both spatially and spectrally, so that coherent lidar systems maintain near immunity from the effects of background light.
The LO also provides a stable reference frequency to allow very precise velocity determination; as a consequence the Doppler shift measurement by a coherent lidar system is inherently calibrated. Finally, the LO amplifies the signal via the beating process to allow operation at a sensitivity that approaches the shot-noise (or quantum) limit. This very high sensitivity permits the operation of coherent systems in an unseeded atmosphere, relying only on detection of weak backscattering from natural aerosols.
To approach theoretical performance limits for ZephIR lidar, an "all-fiber" system that uses optical fiber rather than free-space mirrors for simplified alignment and improved robustness, the detection process must be dominated by shot noise from the LO beam. The laser must not generate significant amplitude noise, placing stringent requirements on its relative intensity noise (RIN) performance. While it is not strictly necessary for the beam to retain coherence over the round-trip, noise generated by interference between parasitic reflections in the optical fibers leads to more stringent laser linewidth requirements.
Wind speeds are derived from the lidar signals that originate from backscattering of the beam by particles in the atmosphere. Generally consisting of dust, organic matter such as pollen, soot, or water droplets, the particles must provide sufficient signal for Doppler analysis and it is assumed their motion faithfully follows that of the wind flow.
The range at which the wind is detected is defined by focusing the beam at the desired distance. ZephIR systems in ground operation measure at ranges from 10 m out to 300 m, but crucially closer ranges are easily achieved, down to just a few feet in front of the ZephIR. These are preferred for better turbine control, as the wind field can evolve as it propagates toward the turbine. The ability to measure at such close distances is a unique property of CW lidar systems.
The standard ZephIR uses a simple conical scan approach with a single beam, taking measurements every 20 ms for dense sampling around the circular 360° path sweep of the turbine's rotor blades. But there are other variants of ZephIR such as the WindScanner, developed in partnership with Risø DTU (Roskilde, Denmark), that uses three scanned beams for rapid interrogation of complex flows.2
ZephIR lidar systems have a continuous, circular scan pattern, gathering 50 line-of-sight Doppler readings around the scan each second (see Fig. 2). When staring horizontally from the nose of a wind turbine, wind speed measurements enable accurate feedforward inputs into the turbine control system, allowing the turbine yaw and collective and individual pitch control to be adjusted for optimal energy extraction, speed control, and fatigue and peak load reduction. "For a well-maintained turbine, energy yield enhancements predicted by academic institutions are currently estimated to be relatively modest, in the range of 1% to 2%," says Woodward. "But a potentially more significant benefit of CW lidar is for mechanical load reduction to increase turbine lifetime."Because ZephIR is an all-fiber system, variants of the standard nacelle-mounted design include blade-mounted systems. Here, the central CW light source feeds optical fibers connected to small, lightweight telescopes embedded in the blades of the turbine for more direct optimization of blade angle-of-attack and other pitch parameters. ZephIR units have also been mounted in the hubs (or spinners) of turbines for unobstructed views of the incoming wind in lidar-ready turbine designs.
Pulsed lidar
Compared to CW lidar where light is continuously transmitted to the atmosphere and the backscattered signal has constant average power from all distances at the same time allowing for constant sensitivity (availability) at all ranges, pulsed lidar systems emit light for a period of time corresponding to the pulse length with differing sensitivities (availabilities) at different ranges. Precision timing circuits then isolate the returned signals to a period of time that corresponds to a specified segment of radial distance along the beam called a range gate. The backscattered signals contained within each gate are then processed to derive the radial velocities along the path of the lidar beam.
"For wind turbine applications, CW lidar needs a mechanical refocus of the telescope lens to select a different range, while pulsed lidar does this through a different time-of-flight selection for the returned light pulses; thus, contrary to CW, pulsed lidar does not necessitate moving parts [which improves reliability]," says Samuel Davoust, scientific product manager at Avent Lidar Technology.
Founded in 2009 by atmospheric lidar technologies company Leosphere (Orsay, France) and NRG Systems (Hinesburg, VT), a manufacturer of wind measurement systems with more than 20 years of experience in turbine-mounted systems, Avent Lidar Technology manufactures the nacelle-mounted, pulsed-Doppler Wind Iris system (see Fig. 3). Using a 1540 nm CW source and a pulsed amplifier, Wind Iris employs a fixed-optics design to gather wind speed and direction at 10 ranges from 40–400 m every 0.1 s (10 Hz sampling). With a wind speed range of -10 (wind moving away from the turbine) to +40 m/s, measurements are sampled over a 15° to 30° half angle or over a custom 3D geometry with a proven accuracy of 0.1 m/s."The face of the turbine is basically a vertical plane and to characterize the approaching wind it is important that the data is in a vertical volume," says Fred Belen, VP for technology and product line manager at Catch the Wind (CTW). "Our second-generation OCS [optical control system] on our pulsed Doppler Vindicator lidar system takes a thousand snapshots of the wind every second and provides a statistical view of the volume at 1–10 Hz. The reason we measure simultaneously at a 1 kHz data rate is because the medium is constantly moving and changing. Measurements not taken simultaneously will always have issues with both spatial coherence [that all Doppler systems struggle with] and temporal coherence [that CW and sequential Doppler systems struggle with]."
Catch the Wind produces laser Doppler velocimeters using commercially available telecom-grade optics, with more than 100,000 hours of operation on wind turbines from a wide range of manufacturers. Using a 1.550 nm eye-safe pulsed laser and fixed mono-static telescope optics, their Vindicator lidar family yields 2D wind speed and direction to control the yaw and collective pitch control (adjusting the blade pitch of all blades simultaneously) of a turbine, while systems with three or more beams can generate 3D information and estimate shear, which is critical for advanced turbine control, including intelligently controlling the pitch of each blade individually.
All lidar techniques rely upon, to some extent, coherence between measurements to compute the wind vector math. "We use a kilohertz-class pulse rate simultaneously on all beams, allowing the OCS to rapidly and independently measure wind data across multiple gates and ranges," says Belen. "Compared to scanning CW lidar or sequentially pulsed lidar, this simultaneous approach eliminates the need to assume temporal coherence for the time required between measurements in the dynamic wind flow. The result is very high data rate and a unique ability to provide nearly real-time information to the control system."
Catch the Wind's fixed-optics design eliminates the need for field adjustment or calibration, and uses telecom-grade distributed feedback (DFB) lasers and a standard two-stage optical amplifier pumped with commercial-grade pump lasers. Belen adds, "We also use some tricks to avoid nonlinear effects in the fiber amplification stage that might broaden the outward bound wavelength of the laser."
The bottom line for wind-turbine lidar is its ability to improve wind energy production. "We have now shown—on multiple turbine types—power increases of greater than 10%," says Belen. "We've really worked hard to understand turbine control and exactly how much more power can realistically be produced. While some of that work falls into the 'secret sauce' category, certain turbine control algorithms lead to substantial optimization of output power." Belen concludes, "The second piece of the economic equation is a bit more difficult to quantify, and that is simply that a turbine pointed more accurately into the wind will see reduced dynamic stress and wear-both power increases and reduced maintenance are important to the wind energy industry."
REFERENCES
1. D. Schlipf et al., "Prospects of Optimization of Energy Production by LiDAR Assisted Control of Wind Turbines," EWEA 2011 Conf., Brussels, Belgium (March 2011).
2. www.yourwindlidar.com/products/windscanner
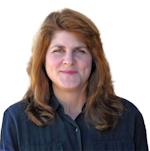
Gail Overton | Senior Editor (2004-2020)
Gail has more than 30 years of engineering, marketing, product management, and editorial experience in the photonics and optical communications industry. Before joining the staff at Laser Focus World in 2004, she held many product management and product marketing roles in the fiber-optics industry, most notably at Hughes (El Segundo, CA), GTE Labs (Waltham, MA), Corning (Corning, NY), Photon Kinetics (Beaverton, OR), and Newport Corporation (Irvine, CA). During her marketing career, Gail published articles in WDM Solutions and Sensors magazine and traveled internationally to conduct product and sales training. Gail received her BS degree in physics, with an emphasis in optics, from San Diego State University in San Diego, CA in May 1986.