PHOTONIC FRONTIERS: DIGITAL HOLOGRAPHY: Digital techniques render real-time response in holography
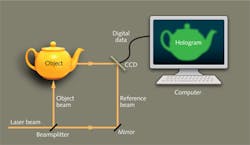
Holography was born as an analog technology, and the development of laser holography by Emmett Leith and Juris Upatnieks drew heavily from Leith's earlier work on optical signal processing, a form of analog computing. Holograms were long recorded on special photographic emulsions applied as coatings to glass plates or film.
The idea of computer generation of holograms dates back to 1966, but that technology long was limited by practical issues of computing capacity, and early computer-generated holograms often were recorded on photographic media. Now a new generation of digital technology has replaced photographic media for recording holograms, and has created new options for processing and displaying holographic images. As in photography, the digital approach offers important advantages, including real-time response, and convenient processing and storage. Applications include 3D microscopy, displays, and video or "telepresence."
Basic computing concepts
Holography is based on recording both the amplitude and phase of the wavefront of light from an object. This is done by combining the wavefront of light from the object with a coherent reference beam from the same source to produce an interference pattern, which is called a hologram. Illuminating the recorded hologram with an identical reference beam reconstructs the original wavefront, which the eyes perceive as showing the original object as if it were present.
In Dennis Gabor's original concept, the object and reference beams followed the same path, and the object itself was two-dimensional. This approach, called on-axis holography, is easy to implement and is still used for some applications, but it is inherently limited to small objects and produces troublesome twin images that overlap. Leith's invention of off-axis holography, in which the object and reference beam follow separate paths, allowed holography of larger objects and removed the twin image from the reconstructed scene.
Computer-generated holography used computers to calculate the interference pattern that a virtual object would produce. That computer-generated hologram was then printed, often on photographic media, and illuminated with a reference beam that produced a 3D image of the virtual object.
The new digital holography replaces the analog photographic film or plate used to record early holograms with a digital detector array that records the hologram, as shown for off-axis holography in Fig. 1. The resulting digital version of the hologram then goes to a computer for further processing.
One possibility is using the computer to extract phase and intensity data from the digitized hologram and further process that data to create a 3D digital model of the original object viewable on a computer display. Alternatively, the digitized hologram can be reproduced on a digital display or a photorefractive material, which is illuminated by a reference beam to optically reconstruct a 3D image. Images can be recorded and displayed singly, as fixed images, or in sequence to produce holographic videos, movies, or telepresence.
How is digital holography used in microscopy?
Microscopy has been a particularly successful application for digital holography, a reminder that Gabor had an impressively sharp view of the future when he invented holography as a way to improve the electron microscope. Digital holography works well for living cells, which can be difficult subjects for conventional microscopy because they are soft and have little natural color contrast. Holography, like phase-contrast microscopy, can record refractive-index differences, which can distinguish cellular components.
For microscopy, the digital hologram is recorded electronically then the resulting digital data usually are processed in real time to create a 3D model for display on a computer screen, as shown in Fig. 2. This method avoids time-consuming photographic processing and allows recording of a time series of images to study dynamic effects. Moreover, writes Myung Kim of the University of South Florida (Tampa, FL), "the complete and accurate representation of the optical field as an array of complex numbers allows many imaging and processing capabilities that are difficult or infeasible in real-space holography."1 Image-processing options include focusing on the object at various distances, quantitative phase-shift measurements, and digital manipulations such as aberration corrections. Digital processing also can suppress the troublesome twin images produced by in-line Gabor holography.A key advantage of digital holography, first demonstrated in 2003, is that it can study living cells without staining, labeling, or otherwise affecting them. This "makes it possible to easily measure cell properties that previously have been very difficult to study in living cells, such as cell thickness, volume, and cell refractive index," write Kersti Alm of Phase Holographic Imaging (Lund, Sweden) and colleagues in a review paper.2 Real-time digital processing permits a series of observations to monitor changes, and the recorded holographic data can be reprocessed to better compare different views.
Digital holographic microscopy does have its limits. A major one is the relatively low spatial frequency response of digital cameras, which limits resolution of reconstructed holograms, write Damien Kelly of the National University of Ireland (Maynooth, Ireland) and colleagues.3 That arises from the spacing of detector elements and their larger size than the grains in photographic media. But Kelly says that new processing techniques promise improvements.
Digital hologram displays
A digitally recorded hologram also can be reconstructed by displaying it on a spatial light modulator (SLM) or photorefractive medium and illuminating it with a reference beam. Diffraction of the input light by the displayed hologram produces a 3-D image, as shown in Fig. 3.Producing a good-quality holographic image requires digital processing of the recorded hologram that takes into account both the illuminating wavelength and the physical characteristics of the display device. That could best be done by transmitting holograms in a standard interchange format and doing the final processing at the device, to account for its particular features, writes V. Michael Bove of the MIT Media Laboratory (Cambridge, MA) in recent review of digital display holography.4 "An ideal SLM would be able to control both the intensity and the phase of light, but given that most practical SLMs can affect only one or the other, a phase-only modulator is more desirable as it is theoretically over five times more diffraction-efficient ... than an amplitude-only modulator." Both types have been demonstrated.
Another advantage of SLMs is that the displayed hologram can be changed dynamically, changing the way it diffracts light. This allows their use as dynamic holographic optical elements for applications requiring adjustable optics, such as generating a conjugate wavefront for adaptive optics, or beam directors for (holographic) optical tweezers. Although the space-bandwidth product of the digital devices is smaller than those of analog holographic materials, with suitable processing liquid-crystal displays and digital micromirrors developed for mass-produced consumer products can be used for many applications, writes Tobias Faist of the University of Stuttgart (Stuttgart, Germany).5
Holographic television or telepresence
The next step beyond dynamic digital holography is holographic television or telepresence. The idea is to record digital holograms at a video-like frame rate, transmit them, then display them in their full three-dimensional glory at the same frame rate.
So far, the most impressive "holographic" demonstrations have not involved any real holograms. The famed "hologram" of Princess Leia pleading for help in Star Wars: Episode I in 1977 was a nonholographic special effect. A series of recent demonstrations of "holographic telepresence" including futurist Ray Kurzweil and long-dead rapper Tupac Shakur were also fakes, based on the "Pepper's Ghost" illusion, which projects a 2D image onto a clear screen on stage.
Holographic motion pictures or television has been a dream since the 1960s, but years of development with analog technology faded away in the mid-1990s. In the past few several years, digital real-time holography has made major progress. "Capture and computation are proving not to be the barriers that people have been assuming they would be," says V. Michael Bove of the MIT Media Laboratory. "The display itself is the limiting factor." Developers are using inexpensive cameras to capture images in incoherent light, then using computers to generate the holograms, with much of the computation done at the display.
The results have included some eye-catching demonstrations. Nasser Peyghambarian of the University of Arizona (Tucson, AZ) and colleagues in 2010 demonstrated telepresence at one frame per second using a new photorefractive material.6 In 2011, Bove's group at MIT hacked the gesture- recognition camera from a Microsoft Kinect to generate input images, which they processed with imaging chips and displayed using a custom-built acousto-optic projector, showing graduate student Edwina Portocarrero dressed up as Princess Leia (see Fig. 4).Outlook
Digital technology has brought big improvements to holographic imaging. Digital imaging and processing continue their relentless advance, and Kim is optimistic that thanks to such new technology and improving pixel resolution, "new holographic imaging capabilities yet to be conceptually imagined will emerge."
The progress of real-time holographic video also has been impressive, but tough problems remain. The resolution remains more like that of early electronic television demonstrations than those of Apollo-era color television broadcasts. Don't expect to keep that old analog CRT television set hooked up to a digital converter and a VCR working long enough to replace it with holovision.
REFERENCES
1. M.K. Kim, "Principles and techniques of digital holographic microscopy," SPIE Reviews, 1 (2010);doi:10.1117/6.0000006.
2. K. Alm et al., "Digital Holography and Cell Studies," Holography, Research and Technologies, InTech, Rijeka, Croatia (2011).
3. D.P. Kelly et al., "Resolution limits in practical digital holographic systems," Opt. Eng., 48, 9, 095801 (September 2009).
4. V. Michael Bove, Jr., "Display Holography's Digital Second Act," Proc. IEEE, 100, 4, 918 (April 2012).
5. S. Zwick, T. Haist, M. Warber, and W. Osten, "Dynamic holography using pixelated light modulators," Appl. Opt., 49, F47 (Sept. 1, 2010).
6. J. Hecht, "Holographic telepresence approaches real-time operation," Laser Focus World, 46, 12 (December 2010).
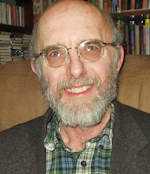
Jeff Hecht | Contributing Editor
Jeff Hecht is a regular contributing editor to Laser Focus World and has been covering the laser industry for 35 years. A prolific book author, Jeff's published works include “Understanding Fiber Optics,” “Understanding Lasers,” “The Laser Guidebook,” and “Beam Weapons: The Next Arms Race.” He also has written books on the histories of lasers and fiber optics, including “City of Light: The Story of Fiber Optics,” and “Beam: The Race to Make the Laser.” Find out more at jeffhecht.com.