PHOTONICS APPLIED: ROBOTICS: Microrobots slide, crawl, and swarm into new applications
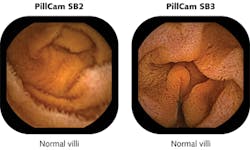
Loosely defined as miniature robots on the order of 1 mm to a few centimeters in size, microrobots or microbots are being developed for a host of new applications that only ultrasmall devices can tackle. For example, 2-mm-long oxygen-sensing microbots injected into the eye can deliver real-time oxygenation information to surgeons; the decades-old pill camera and other robotic capsule endoscope designs have improved vision capabilities and are gaining traction with robotic articulation for improved gastrointestinal analysis; and centimeter-scale crawling and flying microbots could seek signs of life in collapsed buildings or mines.
Microrobotics should be distinguished from nanorobotics. Nanorobots or nanobots are at least an order of magnitude smaller (nanometer-scale) than microrobots and lack the more complex, system-based imaging/actuation/sensing capabilities of microbots. Nanoscale molecular machines are also classified in the nanorobotics category. This article will focus on microrobots only—the nanorobots will have to wait their turn.
Inside the eye
Researchers at the Institute of Robotics and Intelligent Systems at ETH Zurich (Zurich, Switzerland) recognize that for in vivo biomedical tissue diagnostics, smaller and much-less-invasive microrobotic systems can have marked advantages over bulky external equipment such as optical fiber probes and standoff imaging/microscopy systems. "Due to the invasive nature of available methods, the number of studies on humans is limited regarding the effects of inadequate oxygen supply [retinal hypoxia] that is correlated with major eye diseases such as diabetic retinopathy, glaucoma, and age-related macular degeneration," says ETH Zurich scientist Olgaç Ergeneman. "Our intraocular microrobots are tackling this deficiency through real-time oxygen sensing."1
Consisting of magnetically sensitive 2-mm-long oval or cylindrical-shaped platforms coated with an oxygen-sensitive fluorescent dye, the microrobots—which luminesce when illuminated—are magnetically positioned within an aqueous environment. For the specific dye used, luminescence quenching as a function of oxygen level decreases the emission lifetime; a custom-made optical readout system measures this quenching to reveal location-specific oxygen levels within the eye. Ergeneman adds that ETH Zurich has developed and tested magnetically actuated helical micromachines roughly 9 μm long and 2 μm wide capable of controlled swimming and small cargo transport.2 These helical swimmers could eventually become wirelessly controlled platforms for in vivo diagnostics and/or drug delivery in a variety of biological environments.
Along the GI tract
As I researched the capabilities of these retinal microrobots, visions from the 1966 science fiction film Fantastic Voyage come to mind. Essentially, a diagnostic laboratory has been shrunk down to a size that enables insertion into a small surgical opening in the eye. Incredibly, the PillCam from Given Imaging (Yoqneam, Israel) and other robotic capsule endoscope (RCE) designs take microrobotics a step further by adding vision to their repertoire: these swallowable, clinically administered, and commercially available capsules or pills (roughly 11 × 26 mm in size) take pictures as they traverse the human gastrointestinal (GI) tract. Already proven in esophageal, colon, and especially small-intestine disease diagnosis, RCEs with advanced means of locomotion, drug delivery, and even biopsy capability are in the works.
Introduced back in 2000, the PillCam has been used on more than 1.7 million patients and is the subject of 1900 clinical studies. Like all untethered RCEs, PillCam is a battery-powered, wireless-data-transmission imaging device (swallowed by the patient) that traverses the GI tract through natural peristaltic motion in about nine hours.
PillCam SB, developed to visualize the entire small bowel, is now in its third generation as PillCam SB 3, which has 30% higher resolution than the previous generation PillCam SB 2 and incorporates adaptive frame-rate technology that automatically increases the frame rate from 2 frames/s up to 6 frames/s as transit speed increases (see Fig. 1). A miniature white LED emitting from 450 to 700 nm consumes only a few milliwatts to illuminate a 3000 mm2 section of GI tissue in a 150° field of view using a six-part lens assembly only 3 mm thick. With imaging resolution down to 0.07 mm, PillCam SB is CE-marked, available in more than 75 countries, and is pending U.S. FDA 510(k) clearance.
Professor of pathology Guillermo J. Tearney and colleagues at the Wellman Center for Photomedicine at Massachusetts General Hospital (MGH; Boston, MA) have developed an ingestible multivitamin-sized imaging capsule on a fiber-optic tether with advantages over the PillCam and traditional endoscopes (see Fig. 2).3 "We can screen for pre-cancerous Barrett's esophagus or other respiratory/gastrointestinal diseases using the imaging capsule without requiring patient sedation, a hospital setting, or even a trained physician," says Tearney. "And we obtain three-dimensional [3D] microscopic images compared to the 2D macroscopic images acquired by the PillCam and even high-resolution endoscopy."The MGH imaging capsule uses optical frequency-domain imaging (OFDI) technology developed at MGH: a near-infrared laser beam sweeping from 1250 to 1380 nm is first sent to a beamsplitter, where a small portion is captured by a detector as the reference signal and the rest is emitted as a focused, full-width half-maximum, 30-μm-diameter beam from an optical fiber tip (125 μm diameter with 9 μm core) within the capsule rotating at 20 Hz to illuminate an approximate 40 mm circumference section of tissue surrounding the capsule. The reflected signals from the tissue (as a function of depth) are compared to the reference signal and the difference is used to construct a microscopic cross-section that is stacked together with adjacent cross-sections as the capsule moves to form a 3D image. Imaging time for a 15 cm esophageal section with 30 μm (lateral) and 7 μm (axial) resolution takes only 58 seconds.
In addition to PillCam and the MGH capsule, other commercially available RCE devices include the EndoCapsule from Olympus (Tokyo, Japan), the MiRo capsule from IntroMedic (Seoul, South Korea), and the OMOM capsule from Jinshan Science and Technology Group (Chongqing, China).4 OMOM, though slightly larger than PillCam at 27.9 mm long and 13 mm in diameter, offers an adjustable image format, white-balance control, variable frame-rate sampling frequency and illumination intensity, and automatic or manual exposure.
Besides the ongoing imaging improvements for RCEs, the InteliSite capsule from Innovative Devices (Raleigh, NC), the Enterion capsule from Phaeton Research (Nottingham, England), and the MAARS capsule from Matesy (Jena, Germany) are also addressing drug delivery and biopsy through various diffusion-based or spring-actuated methods. In their most recent work, Vanderbilt University (Nashville, TN) professors Robert J. Webster III and Pietro Valdastri are attempting to overcome one weakness of RCE compared to traditional endoscopy, which is RCE's inability so far to "inflate" the gastrointestinal tract wirelessly—"wireless insufflation"—to obtain better images of its normally folded surface.5 To date, their experiments with a capsule that mixes potassium bicarbonate powder with citric acid that escapes through small holes in the 3D printed capsule have been successful in generating the required carbon dioxide to sufficiently insufflate a 5.5 cm diameter porcine colon.
Webster and Valdastri were also part of a team from Vanderbilt University and Scuola Superiore Sant'Anna (Pisa, Italy) that developed a truly robotic-looking imaging capsule with physical appendages that was designed to crawl along the esophageal or gastrointestinal tract (see Fig. 3).6 Unfortunately, the device development was short-lived: "Although the 12-legged mechanics of our capsule—comprised of more than 70 high-precision components—enabled high foot force [0.63 N average propulsive force at each leg tip] to traverse slippery, tubular environments, the device became bogged down with biological tissue and eventually stopped working," says Valdastri, who is also principal investigator at Vanderbilt's Science and Technology of Robotics in Medicine (STORM) Lab."The robotic platform for colonoscopy we are currently working on at the STORM Lab is based on closed-loop magnetic guidance of a soft-tethered endoscopic capsule using a remote seven-degree-of-freedom robotic arm. All the major developers of capsule endoscopes like Given Imaging and Olympus are moving in the same direction and magnetic platforms to control wireless ingestible cameras are concretely the next revolution in the field of gastrointestinal endoscopy."7 There is even an untethered soft-capsule robot from Carnegie Mellon University (Pittsburgh, PA) that can be rolled inside the stomach using remote magnetic actuation.8 This soft capsule offers noninvasive stomach imaging, drug delivery, and can even conduct biopsy and elastography by a doctor's direct control.
Beyond magnetics, other means of mobility being explored for RCEs include biomimetic robotic feet fitted with photolithographically patterned silicon pads from the University of Leeds that mimic the "sticky" feet of tree frogs for motion inside the abdomen (see http://youtu.be/XTrogpss6W0) and micro-patterned polydimethylsiloxane (PDMS) treads from the University of Colorado (Boulder, CO).9,10 The treads—driven by a gear train and a direct-current motor within the tethered RCE to travel at speeds of 3 mm/s—are patterned with 140-μm-diameter, 70-μm-high pillars spaced 245 μm apart (to simulate microhairs on the feet of insects) and fabricated through micromolding using a template containing tens of thousands of laser-drilled holes (see Fig. 4).Into harm's way
Much like the large robots that are sent into harm's way to detonate a bomb, smaller-sized microrobots with a variety of sensing capabilities are being developed to crawl, fly, or essentially "swarm" in large numbers into a variety of dangerous situations such as mining disasters, earthquakes, and chemical spills, or for applications in environmental sensing and industrial research. Jasmine swarm robots (www.swarmrobot.org) were originally developed around 2005 on a roughly 3 cm3 platform by engineers at Karlsruhe Institute of Technology (KIT; Karlsruhe, Germany) and the University of Stuttgart (Stuttgart, Germany). Since then, Jasmine has been succeeded by Wanda microrobots, and there is even a 10X smaller I-SWARM robot ( i-swarm.org) that measures just 3 mm3 (see Fig. 5).The goal of swarm robots, as summarized in the I-SWARM Sixth Framework Programme project (http://rob.ipr.kit.edu/english/540_651.php) from which I-SWARM originated, is to mass-produce microrobots that can be deployed as a swarm consisting of up to 1000 robot clients equipped with limited on-board intelligence with different sensors, manipulators, and computational power that could perform a variety of functions such as microassembly, biological, medical, or cleaning tasks.
The Jasmine and I-SWARM microrobots are currently open-source platforms; consequently, detailed schematics of circuit-board design and mechanics are available online. Though small in size, these tiny microbots pack in a lot of technology. For I-SWARM, communication between microbots is possible at 1 bit/s at 10 mm distances using a simple LED/photodiode send/receive sensor; locomotion is accomplished by a flexible printed circuit board with three legs beneath a piezoelectric polymer actuator multilayer film; a vibrating cantilever sensor allows the microbot to sense objects in its path; and swarm mathematics and software are used to enable "autonomous" robotic movement using GPS or light-based signaling.
Unfortunately, there are more microrobotic laboratories doing inspiring work than I am able to cover in this Laser Focus World feature. Be sure to investigate Berkeley's Biomimetic Millisystems Lab and the University of Maryland's Micro Robotics Lab, and don't forget to view the images and see the videos from the Harvard Microrobotics Laboratory, which is developing biologically inspired, insect-scale crawling and flying robots (see http://youtu.be/b9FDkJZCMuE) such as RoboBees that incorporate microelectromechanical systems for articulation and actuation and ambulatory robots that resemble centipedes with soft, artificial sensing skin. In the not-too-distant future, you might be swallowing a robotic capsule at your next doctor's appointment or calling in a microrobotic swarm of bees to surreptitiously find out what your "remote" employees are up to.
REFERENCES
1. O. Ergeneman et al., IEEE Transact. Biomed. Eng., 59, 11, 3104–3109 (November 2012).
2. S. Tottori et al., Adv. Mater., 24, 6, 811–816 (February 2012).
3. M. J. Gora et al., Nat. Med., 19, 2, 238–240 (2013).
4. P. Valdastri et al., Annual Rev. Biomed. Eng., 14, 397–429 (August 2012).
5. J. L. Gorlewicz et al., IEEE Transact. Biomed. Eng., 60, 5, 1225–1233 (May 2013).
6. P. Valdastri et al., IEEE Transact. Robot., 25, 5, 1047–1057 (October 2009).
7. J.-F. Rey et al., Gastrointest. Endosc., 75, 2, 373–381 (2012).
8. S. Kim and M. Sitti, IEEE Transact. Robot., 28, 5, 1198–1202 (2012).
9. R. Roshan et al., Acta Biomater., 7, 11, 4007-4017 (November 2011).
10. L. J. Sliker et al., Surg. Endosc., 26, 2862-2869 (2012).
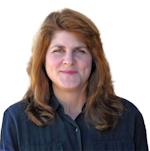
Gail Overton | Senior Editor (2004-2020)
Gail has more than 30 years of engineering, marketing, product management, and editorial experience in the photonics and optical communications industry. Before joining the staff at Laser Focus World in 2004, she held many product management and product marketing roles in the fiber-optics industry, most notably at Hughes (El Segundo, CA), GTE Labs (Waltham, MA), Corning (Corning, NY), Photon Kinetics (Beaverton, OR), and Newport Corporation (Irvine, CA). During her marketing career, Gail published articles in WDM Solutions and Sensors magazine and traveled internationally to conduct product and sales training. Gail received her BS degree in physics, with an emphasis in optics, from San Diego State University in San Diego, CA in May 1986.