Photonic Frontiers: Fiber Lasers: Novel fiber lasers offer new capabilities
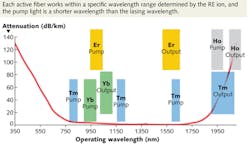
Ytterbium (Yb)- and erbium (Er)-doped fiber lasers have been brilliantly successful in applications from high-power materials to rugged femtosecond sources. Now, different types of fiber lasers are offering new capabilities. Commercial thulium-doped fiber lasers can deliver 200 W continuous wave in the 2 μm band, important for medical and military applications. Holmium-doped fibers can reach atmospheric windows at 2.17 μm.
More developments are in the pipeline. Non-silica fibers can emit longer mid-infrared wavelengths. Novel fiber designs can increase nonlinearity for applications such as optical parametric oscillators (OPOs) or supercontinuum sources, or reduce nonlinearity to increase energy in short pulses.
Fiber laser limitations
Ytterbium and erbium have strong absorption lines that match the output of powerful pump diodes and emit wavelengths where silica fibers have low attenuation. However, they only cover bands near 1030 and 1550 nm, leaving holes in the important 1300 nm range, and at longer wavelengths. Silica absorption becomes problematic beyond about 2.17 μm, so mid-infrared fiber lasers need other host materials.
The tight optical confinement that makes fiber lasers work well also comes at a cost-by concentrating power, it increases nonlinear effects, potentially limiting energy in short pulses. Novel microstructured fiber designs can expand the effective mode area, reducing power density and nonlinear effects. Alternatively, they can compress the effective mode area, increasing nonlinearity for use in OPOs or supercontinuum sources.
The quest for novel fiber lasers thus becomes a search for new materials and fiber designs. One newcomer is bismuth, which has demonstrated CW laser emission of more than a watt and has been observed on several fiber lines between 1150 and 1550 nm, nicely filling the gap between Yb and Er fiber lasers.1
Thulium and holmium fiber lasers
The best-developed of the emerging fiber lasers is thulium, with commercial models available from multiple vendors. The highest power is a 200 W CW version emitting at 1900 to 2040 nm offered by IPG Photonics (Oxford, MA) for industrial applications such as cutting thermoplastics. AdValue Photonics (Tucson, AZ) offers a 1 W single-frequency laser with 50 kHz linewidth, as well as a 250 μJ Q-switched model and a mode-locked laser delivering 35 nJ pulses. CW power in the laboratory has reached kilowatt-class.
Even higher powers have been obtained in the laboratory, with kilowatt-class power reported in 2009 by a team from Nufern2 (East Granby, CT) and by Nufern and Q-Peak (Bedford, MA) in 2010.3 The keys to those high powers were high thulium levels in the doped fiber and pumping with 790 nm laser diodes, says Nufern president Martin Seifert. The diode-pumping scheme uses a quirk of thulium absorption, which allows a single 790 nm photon to excite a pair of adjacent thulium atoms to the upper level of the 2 μm laser band. AdValue uses 790 nm diodes for multimode pumping, and Er-fiber lasers near 1560 nm for singlemode pumping, says company president Shibin Jiang.4
The wavelength range of thulium in silica glass is limited to about 2.05 μm by fiber loss, as shown in Figure 1. That poses a problem because atmospheric transmission is much better at 2.1 μm, a crucial issue for remote sensing and applications that require high-power transmission. The longer wavelengths also suppress potentially loss-inducing Brillouin scattering in the fiber. Some medical applications also benefit from such wavelengths.
This problem led to development of holmium-doped fibers, which emit at longer wavelength when pumped by thulium at 1.95 μm. A team from the Australian Defense Science and Technology Organization (Edinburgh, South Australia) and Nufern recently produced 407 W at 2.12 μm from holmium by cladding.5 Tuning at high power was limited to 2.10 to 2.13 μm, but at lower powers, holmium could be tuned from 2.05 to 2.15 μm.
Non-oxide fiber hosts
Non-silica glasses have much lower losses at wavelengths longer than 1.9 μm so they are being investigated as hosts for mid-IR fiber lasers.
The fluoride glass ZBLAN (ZrF4-BaF2-LaF3-AlF3-NaF) is an attractive host for mid-infrared fiber lasers, with a transmission window from 0.3 to 4.3 μm. A 2011 review lists nine fiber laser lines in ZBLAN between 2 and 4 μm with demonstrated output from 10 mW to over 10 W, as shown in Figure 2. A related indium-fluoride glass has a window extending to 5.3 μm.6 A dysprosium-doped ZBLAN fiber laser produced 90 mW output near 3 μm with slope efficiency to 23 percent when pumped with a 1088 nm Yb-doped fiber laser.7Another attractive material is tellurite (TeO2), which a team at the Shanghai Institute of Optics and Fine Mechanics (Shanghai, China) mixed with 30% tungsten oxide (WO3) and 10% lanthanum oxide (La2O3) to make double-clad fibers. Pumping at 800 nm with laser diodes, the team produced 494 mW at 1.9 μm from a 20 cm length of thulium doped fiber and 35 mW at 2.1 μm from a 7 cm length of fiber co-doped with thulium and holmium.8 Another Shanghai group produced 2.7 μm when pumping a fluoro-tellurite glass co-doped with Er and holmium with 980 nm diodes, but did not report the power.9
Higher powers from femtosecond fiber lasers
New fiber structures are increasing the energy of femtosecond fiber pulses. "Anomalous dispersion and self-focusing nonlinearity dominate pulse shaping in virtually every femtosecond laser in use today," says Frank Wise of Cornell University (Ithaca, NY). Femtosecond fiber lasers' production of solitons stabilizes pulse shape but limits pulse energy to 10 to 100 times lower than in bulk titanium-sapphire lasers.
To overcome that limit, Wise avoids anomalous dispersion by using Yb-doped fibers because silica fibers have normal dispersion at their 1 μm wavelength. Chirping pulses and filtering them to trim the fringes of the spectrum generates "dissipative solitons" which remain stable at roughly 100 times the energy of an ordinary soliton.10 "We can now make femtosecond fiber lasers that can emit 30 nJ pulses and average power of a couple of watts, on par with Ti-sapphire," for use in nonlinear spectroscopy and other applications, says Wise.11 At Photonics West 2014, Kapteyn-Murnane Laboratories (Boulder, CO) introduced a commercial version called Y-Fi, shown in Figure 3, which emits 400 nJ pulses at rates to 10 MHz.Doped photonic crystal fibers (PCF) with very large effective core areas can produce higher average power than Ti-sapphire. A team at the Friedrich Schiller University (Jena, Germany) used a microstructured PCF with an 80 μm core in the gain fiber to produce 0.9 μJ pulses with average power of 66 W.12 Such fibers are stiff and have high losses if bent.
OPOs, Raman fiber lasers, and supercontinuums
Femtosecond fiber lasers can synchronously pump fiber optical parametric oscillators (OPOs). Early versions emitted only modest powers, but in 2013 Khanh Kieu at the University of Arizona showed that chirping pulses and operating at normal dispersion wavelengths could generate 1.45-nJ pulses to produce average power of 60 milliwatts at 1600 nm.13 The researchers coupled pulses from a 1560 nm Er-fiber oscillator into a cavity that included a 24 m length of an Er-fiber amplifier and a 5 m length of dispersion-shifted fiber, producing signal and idler outputs near 1510 and 1610 nm. At CLEO 2014 in June, Kieu's group will report achieving a wide tuning range.
IPG introduced an interesting new twist on Raman fiber lasers at Photonics West 2014: red and orange beams. A Yb-fiber laser pump stimulated Raman scattering in a second fiber, then the IR Raman emission is frequency-doubled into the visible. The concept of doubling Raman output to produce 589 nm goes back several years.14 The commercial orange laser emits up to 15 W CW and is tunable between 570 and 600 nm; a similar red version emits up to 20 W CW at 615 to 650 nm.
Fiber lasers have also put a fresh spin on supercontinuum sources. Dispersion-engineered microstructured nonlinear fibers "significantly relax the requirements for supercontinuum generation," wrote Jihong Geng and colleagues from AdValue Photonics in 2012. By tightly confining the pump light in a small area with low attention, such fibers can efficiently generate a broadband supercontinuum from a compact all-fiber source. They generate a broadband spectrum flat from 1.9 to 2.5 μm with peak power over 15 kW by pumping two superradiative thulium transitions, one at 1.8 to 2.1 μm, the other at 2.2 to 2.5 μm. Adding two short lengths of chalcogenide fiber further enhanced the spectrum.15
Outlook
Fiber lasers have been a remarkable success, and silica has been a remarkably successful host material for them. However, other fiber hosts and continued refinements in fiber structure are still needed.
"The best approach to meet future challenges for high-performance optical fibers" is to focus on glass science, wrote John Ballato of Clemson University (Clemson, SC) and Peter Dragic of the University of Illinois (Urbana, IL) in a recent review arguing for "rethinking optical fiber."16 They wrote about fibers in general, but their argument clearly applies to fiber lasers. They cited particularly the molten-core fabrication process used to make semiconductor-core fibers.17 That approach has yet to be used for fiber lasers, but silicon Raman lasers have already been demonstrated on chips. Anna Peacock of the University of Southampton (Southampton, UK) says "it is easy to imagine [silicon Raman lasers] being transferred to the fiber platform—bringing with it the obvious benefits of the fiber geometry."
But that's not the whole story. Microstructured fibers have already expanded the capabilities of silica, and have promised further improvements. They do have some problems, such as the rigidity and light leakage of large-core fibers, but new materials also have problems. In the long term, we need both better materials and better microstructures to build better fiber lasers.
References
1. I.A. Bufetov, et al., "Bi-doped optical fibers and fiber lasers," to appear in IEEE Journal of Selected Topics in Quantum Electronics; doi: 10.1109/JSTQE.2014.2312926.
2. Adrian Carter, Bryce Samson, and Kanishka Tankala, "Thulium-doped fiber forms kilowatt-class laser," Laser Focus World, April 2009, http://www.laserfocusworld.com/articles/print/volume-45/issue-4/features/fiber-lasers-thulium-doped-fiber-forms-kilowatt-class-laser.html.
3. Thomas Ehrenreich, et al., "1-kW all-glass Tm:fiber laser," http://www.qpeak.com/Meetings/PW%202010%201kW%20Tm_fiber%20laser.pdf.
4. Shibin Jiang, "Two-micron thulium-doped fiber lasers achieve 10-kW peak power," Laser Focus World, 49, 2, 52 (2013).
5. Alexander Hemming et al., "Power scaling of resonantly pumped holmium-doped fiber lasers," Solid-state and Diode Laser Technology Review Technical Digest, (Directed Energy Professionals Society, 2013), paper 8.
6. Mohammed Saad, "Heavy metal fluoride glass fibers and their applications," in Passive Components and Fiber-Based Devices VIII, ed. Bishnu P. Pal, Proc. SPIE, 8307, 83070N (2011); doi: 10.1117/12.915295.
7. Y.H. Tsang and A.E. El-Taher, "Efficient lasing at near 3 μm by a Dy-doped ZBLAN fiber laser pumped at ~1.1 μm by an Yb fiber laser," Laser Phys. Lett., 8, 11, 818 (2011); doi: 10.1002/lapl.201110068.
8. Kefeng Li, et al., "Tm3+ and Tm3+-Ho3+ co-doped tungsten tellurite glass single mode fiber laser," Opt. Express, 20, 9, 10115 (April 2012).
9. Yaoyao Ma et al., "Er3+/Ho3+ Codoped fluorotellurite glasses for 2.7 μm fiber laser materials," Fibers, 2013, 1, 2, 11; doi:10.3390/fib1020011.
10. Frank W. Wise, "Femtosecond fiber lasers based on dissipative processes for nonlinear microscopy," IEEE J. Sel. Top. Quantum Electron., 18, 4, 1412 (2012).
11. C. Xu and F. W. Wise, "Recent advances in fiber lasers for nonlinear microscopy," Nat.Photonics (2013); doi: 10.1038/nphoton.2013.284.
12. Martin Baumgartl et al., "66 W average power from a microjoule-class sub-100 fs fiber oscillator" Opt. Lett., 37, 10, 1640 (2012).
13. T. N. Nguyen et al., "Normal dispersion femtosecond fiber optical parametric oscillator," Optics Lett., 38, 3616 (2013).
14. D. Georgiev et al., "Watts-level frequency doubling of a narrow line linearly polarized Raman fiber laser to 589 nm," Opt. Express, 13, 18, 6772 (2005).
15. Jihong Geng, Qing Wang, and Shibin Jiang, "High-spectral-flatness mid-infrared supercontinuum generated from a Tm-doped fiber amplifier," Appl. Opt., 51, 7, 834 (2012).
16. John Ballato and Peter Dragic, "Rethinking optical fiber: New demands, old glasses," J. Am. Ceramic Soc., 96, 9, 2675 (2013); doi: 10.1111/jace.12516.
17. Anna Peacock et al., "Semiconductor optical fibres: Progress and opportunities," Laser & Photon. Reviews, 8, 1, 53 (2014); doi: 10.1002/lpor.201300016.
About the Author
Jeff Hecht
Contributing Editor
Jeff Hecht is a regular contributing editor to Laser Focus World and has been covering the laser industry for 35 years. A prolific book author, Jeff's published works include “Understanding Fiber Optics,” “Understanding Lasers,” “The Laser Guidebook,” and “Beam Weapons: The Next Arms Race.” He also has written books on the histories of lasers and fiber optics, including “City of Light: The Story of Fiber Optics,” and “Beam: The Race to Make the Laser.” Find out more at jeffhecht.com.