Photonic Frontiers: Solid State Lasers: Looking back/Looking forward: A long way from the ruby laser
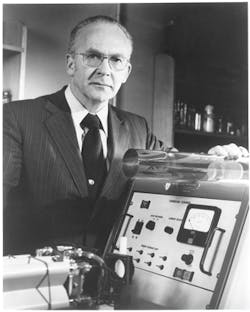
Theodore Maiman's choice of commercial off-the-shelf components allowed ruby to be the first commercial laser. TRG Inc. and Bell Labs reproduced Maiman's design within weeks. In less than a year, ruby lasers were products. Raytheon probably was first to market, showing its first laser at a March 1961 meeting of the Institute of Radio Engineers, a forerunner of IEEE. Maiman started a laser group in early 1961 at a short-lived company called Quantatron, and that group became the nucleus of Korad Inc. (Santa Monica, CA), a major laser maker of the 1960s.
Lloyd Cross founded Trion Instruments in Ann Arbor, MI, in March 1961 with $30,000 from friends and relatives and expanded quickly. One early employee, Clarke Harris, recalls asking "what's a laser?" when he interviewed as a fresh Michigan graduate in physics. Trion hired him nonetheless, and when he started in June 1961, more than a dozen people were at work making and selling lasers. The young company quickly became important in the field; Peter Franken used a rented Trion laser in his milestone demonstration of optical frequency doubling.
Trion faded after Lear-Siegler bought it in 1962, but Harris was hooked and spent the rest of his career in lasers. So did Jim Hobart, who had joined Trion with a fresh Michigan PhD and went on to found Coherent Radiation (now Coherent Inc.). Cross became a noted holographer.
The age of ruby and neodymium
Bell Labs demonstrated the first neodymium laser in calcium tungstate in 1961. Eli Snitzer at American Optical (Southbridge, MA) was close behind with neodymium-glass, and his long thin rod had the neodymium doped into a core surrounded by a lower-index cladding—making it the first fiber laser. In 1964, Snitzer made the first Nd-glass amplifier by winding a coil of doped fiber around a linear flashlamp.
The biggest attraction of Nd glass at that time was the ease of making large rods to generate high-energy pulses, recalls Bill Shiner, who worked for Snitzer. They generated a record 5 kJ from a thick rod like the one shown with Snitzer in Figure 1, but the results were classified for years.
Ruby was the most prominent laser in the first products column in the January 1965 Laser Focus (now Laser Focus World). Optics Technology (Belmont, CA) offered one for $995 ("not including rubies") that emitted a 0.5 J pulse when powered by 400 J input. TRG offered a "man-portable" 30-pound Q-switched ruby rangefinder. In the same issue, Eastman Kodak (Rochester, NY) announced a new ytterbium-doped laser glass, but it didn't catch on because it was hard to pump with a flashlamp.
Solid-state lasers were less prominent when I joined Laser Focus in 1974. The big news was a controversy over fusion yields claimed by KMS Fusion with its big Nd-glass fusion laser (July 1974). A full-page ad in the same issue from Apollo Lasers promoted double-pulsed ruby lasers for holography. The solid-state laser of choice for most applications was Nd-YAG, available both pulsed and continuous wave (CW).
Solid-state revolutions
A decade later, a solid-state revolution was underway. An early sign was a feature in our February 1982 issue by John Walling of Allied Electro-Optical Products (Warren, NJ) that described alexandrite, the first widely tunable solid-state laser. In June 1982, Peter Moulton of MIT Lincoln Laboratory told the International Quantum Electronics Conference that the new titanium-sapphire laser had higher quantum efficiency, gain, and bandwidth than alexandrite. He finally published a detailed analysis in 1986,1 and in 1988 Spectra-Physics (Mountain View, CA) and Schwartz Electro-Optics (Orlando, FL, with a laser division in Concord, MA) introduced the first commercial Ti-sapphire lasers. A Spectra-Physics ad on the back cover of our January 1989 issue said the company's new CW Ti-sapphire laser would mean "goodbye to dye," at least in the near infrared. Ti-sapphire's broader bandwidth also allowed it to generate shorter pulses, pushing ultrashort lasers deeper into the femtosecond regime.A third solid-state revolution was brewing in David Payne's lab at the University of Southampton (UK). In 1985, he found a new way to dope rare earths into a singlemode fiber.3 The researchers initially made sensors, then tried lasers and optical amplifiers. At the Optical Fiber Communications conference (OFC) in 1987, they reported a gain of 26 dB in a 1.55 µm erbium amplifier.4 At OFC 1988, as reported in LFW's Jan. 1988 issue, the researchers reported 20 dB gain across 300 GHz in a transmission test, opening the door to wavelength-division multiplexing (WDM).
Semiconductor optical amplifiers reached the market first, from BT&D Technologies (Wilmington, DE) in 1989. But fiber amplifiers won out because of their broader bandwidth, lower noise, and the development of diode pumping. Payne found an erbium pump band at 980 nm; Snitzer found a second pump band at 1480 nm;5 and Emmanuel Desurvire at Bell Labs measured 37 dB gain on the new line.6 Development of high-power pump diodes brought WDM-capable fiber amplifiers to the market in the 1990s, multiplying the capacity of fiber-optic cables. That brought the irrational exuberance of the internet/telecommunications "Bubble," the tremendous capacity of today's global telecommunications network, and a knighthood for Payne.
Our January 1995 market survey found 1994 solid-state laser sales were dominated by lamp-pumped lasers—nearly 6400 units worth a hefty $200 million. However, sales of diode-pumped lasers exceeded 1400 units and reached $23 million, and 650 Ti-sapphire lasers were sold for $31 million. Laser Focus World's commercial technology achievement award showed the trend, going to Uniphase (San Jose, CA) for a compact 100 mW diode-pumped doubled green laser. The following year's award, announced in January 1996, went to Lightwave Electronics (Mountain View, CA) for a singlemode 2 W diode-pumped green laser-20 times more powerful than the previous year's winner. On the back cover of that issue, Spectra-Physics announced a 5 W diode-pumped green laser that ran on single-phase 110 V power.
Fiber lasers
The power of fiber lasers grew quietly in the shadow of the Bubble mania. In 1996, IPG Photonics (Oxford, MA), together with Polaroid, Spectra Diode Labs, and Spectra-Physics, introduced 10-W diode-pumped ytterbium-doped fiber lasers. IPG reached 100 W in 2000, and in our August 2002 issue IPG founder Valentin Gapontsev described further advances, including IPG's new 2 kW CW industrial fiber laser "for heavy-duty metal-welding applications in the auto industry." The package was 1.18 × 1.1 × 0.6 m.
Fiber lasers had become an important part of the laser market by 2004, with sales reaching 2342 units worth $84 million, our January 2005 issue reported. Lamp-pumped lasers accounted for most of the dollars in solid-state sales, $644 million, but the 15,000 units fell short of the nearly 15,600 bulk diode-pumped lasers, with a total price of $270 million. The same issue reported an impressive technical achievement with Ti-sapphire, producing an intensity of 7 x 1021 W/cm2 with a 30 fs, 1.4-J pulse.
High-power solid-state lasers
In an April 2007 feature, I described the Pentagon's Joint High Power Solid-State Laser (JHPSSL) weapon program that was based on diode-pumped slab lasers. The goal was to demonstrate 100 kW output for 500 s from an electrically powered laser by the end of 2008 for use against rockets, artillery, and mortars. Northrop-Grumman (Redondo Beach, CA) reported their success in March 2009. The same year the Lawrence Livermore National Laboratory completed the National Ignition Facility (NIF), which program director Ed Moses called "the biggest and most complex optical instrument ever built," as well as the world's highest-energy laser. A November 2009 feature reported plans to ramp ultraviolet pulse energy slowly to the full 1.8 MJ level, aiming to ignite fusion plasmas by 2012. In 2010, I toured NIF while attending Photonics West, and was awed by its scale and complexity. I also was amazed to be able to buy a doubled green laser pointer for $15 at the show.NIF reached 1.8 MJ in March 2012 and had fired 1100 shots by the end of the year. However, in February 2013, we reported it had failed to reach ignition. The laser met specifications, but experiments are continuing to understand why the plasma physics did not cooperate.
Industrial fiber lasers are now used in some laser-weapon demonstrations. Output from six industrial fiber lasers feed into a single beam director in the Navy Laser Weapon System (LaWS), deployed on the USS Ponce in the Persian Gulf in the summer of 2014, where it successfully detonated targets (see Fig. 4).
Fiber lasers now lead industrial laser sales, our January 2015 market review reported. Fibers account for $960 million of the $2.62 billion in 2014 industrial laser sales, ahead of carbon dioxide with sales of $874 million and bulk solid-state with sales of $444 million (including both diode and lamp-pumped). Back in 2004, fiber lasers were a distant fifth, accounting for only $56 million of total $1.467 billion, with CO2 the leader at $597 million.
The future of solid-state lasers
Fiber lasers also hold the record for output power, a 100 kW CW laser that IPG delivered to Osaka University for materials-working research. "In theory, if you have enough money you can get all the power you want from fiber lasers," says Bill Shiner, now VP for industrial lasers at IPG. The 100 kW beam comes from a 300 µm core, he says, so a 500 µm core might generate a 200 kW beam.Wall-plug efficiency continues to improve. It has reached 45%, and in time Shiner expects all fiber lasers to reach that level. The combination of high power and efficiency will open new applications. "3D manufacturing in metals would not happen without singlemode fiber lasers," he says (see Fig. 5).
"Fiber is phenomenal as a platform," says Bill Krupke, a former Livermore laser researcher who consults on laser development. Fiber lasers are so efficient they can pump nonlinear optical systems or new solid-state infrared sources. "People are starting to request kilowatt-class green lasers," he says. At wavelengths below 400 nm, he adds, "what's going to dominate going forward is the emergence of new pump sources."
Krupke also is fascinated by the potential of nanolasers, which are still in the early stages. He mentions "core-shell" spherical devices in which an inner light emitter excites a thin-film shell doped with rare earths. Such devices might activate individual neurons, or deliver light precisely to cells for sensing applications.
More will doubtless follow and bring us new generations of solid-state surprises.
REFERENCES
1. P. F. Moulton, "Spectroscopic and laser characteristics of Ti:Al2O3," J. Opt. Soc. Am. B 3, 1, 125 (1986).
2. R. J. Keyes and T. M. Quist, "Injection luminescent pumping of CaF2:U3+ with GaAs diode lasers," Appl. Phys. Lett. 4, 3, 50 (1964).
3. S. B. Poole, D. N. Payne, and M. E. Fermann, "Fabrication of low-loss optical fibres containing rare-earth ions," Electron. Lett. 21, 737 (1985).
4. R. J. Mears et al., "High-gain rare-earth doped fiber amplifier at 1.54 µm," paper WI2 in Optical Fiber Communication, 1987 OSA Technical Digest Series, paper WI2.
5. E. Snitzer et al., "Erbium fiber laser amplifier at 1.55 µm with pump at 1.49 µm and Yb sensitized Er oscillator," in Optical Fiber Communication, Vol. 1 of 1988 OSA Technical Digest Series, paper PD2.
6. E. Desurvire et al., "Efficient erbium-doped fiber amplifiers at a 1.53-µm wavelength with a high output saturation power," Opt. Lett. 14, 1266 (1989).
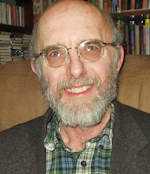
Jeff Hecht | Contributing Editor
Jeff Hecht is a regular contributing editor to Laser Focus World and has been covering the laser industry for 35 years. A prolific book author, Jeff's published works include “Understanding Fiber Optics,” “Understanding Lasers,” “The Laser Guidebook,” and “Beam Weapons: The Next Arms Race.” He also has written books on the histories of lasers and fiber optics, including “City of Light: The Story of Fiber Optics,” and “Beam: The Race to Make the Laser.” Find out more at jeffhecht.com.