Looking back/Looking forward: Gas lasers: Durable survivors evolve new forms
Gas lasers began with the helium-neon (He-Ne) laser, which took Ali Javan, Bill Bennett, and Don Herriott more than two years to develop at Bell Labs, and initially emitted at 1153 nm. In May 1962, Alan White and Dane Rigden took a crucial step by making the first 632.8-nm red He-Ne. It was the first visible continuous-wave (CW) laser, and by 1963 Spectra-Physics (Mountain View, CA) was making them in a partnership with Perkin-Elmer.
Several companies were producing red He-Ne lasers when Laser Focus began publication on January 1, 1965. The technology was young, and it took a long laser to make a milliwatt. A 1 mW He-Ne from Optics Technology Inc. (Belmont, CA) was 47 inches long and sold for $2495 ($300 more than a new Chevrolet Malibu in 1965). A 3 to 5 mW model from H-NU Systems (Palo Alto, CA) sold for $1100 and had a 150 cm tube.
Holography makes headlines
Our first issue also heralded the first three-dimensional holograms, made by Emmett Leith and Juris Upatnieks at the University of Michigan (Ann Arbor) with red He-Ne lasers. Our story spanned several pages and showed a now-iconic image of a holographic toy train (see Fig. 1).Carbon-dioxide lasers led the power race. Our November 15, 1965 issue reported that Perkin-Elmer had set records for CW output power at 130 W and efficiency at 13.8%. Our December 15, 1965 issue reported another record: quasi-CW output of 183 W achieved by the inventor of the CO2 laser, C. Kumar N. Patel of Bell Labs.
Holography fascinated founding editor Bill Bushor, who included holograms from PTR Inc. (Waltham, MA) in all 9000 copies of the April 1967 Laser Focus, the first holograms included in a magazine. Among the many others fascinated by holography was my undergraduate advisor at Caltech, Nick George, with whom I spent a summer in his holography lab.
Gas lasers dominate the market
That experience helped me land a job at Laser Focus a few years later. Once there, I saw that gas laser ads filled the July 1974 issue. Coherent Radiation (Palo Alto, CA) advertised ion lasers on the inside front cover; Spectra-Physics advertised them on the back cover; and Metrologic (Bellmawr, NJ) advertised a He-Ne alignment laser on the first page (see Fig. 3). Inside ads included ion lasers from Lexel (Palo Alto, CA); 50-1000 W CW CO2 lasers from Photon Sources (Livonia, MI); He-Ne lasers and helium-cadmium lasers from Liconix (Mountain View, CA); He-Cd from RCA (Lancaster, PA); and nitrogen lasers from the National Research Group (Madison, WI).Some news looks unusual in retrospect. A December 1974 story told of two gas lasers pumped by neutrons from pulsed nuclear reactors at Sandia National Laboratories (Albuquerque, NM) and at the Los Alamos National Laboratory (NM). Jack Walker of Sandia predicted that in future versions of the lab's helium-xenon laser "more than 50% of the fission energy...could be converted into laser energy," although the demonstrated efficiency was under 1%.
Gas lasers accounted for over half the $70.8 million in sales predicted in our January 1975 market report. Projected 1975 sales were $19.8 million for CO2, $10 million for He-Ne, $6.5 million for ion, and $0.9 million for He-Cd. The February 1975 cover showed a 6-kW CO2 laser from United Aircraft's Hamilton-Standard Division welding an auto underbody at a Ford Motor Company plant in Dearborn, MI.
That year also saw key steps toward practical rare-gas halide excimer lasers. Our December 1975 issue reported the first discharge pumping of xenon fluoride at 351 and 353 nm at the Naval Research Laboratory (Washington, DC). Lead author Ralph Burnham of Science Applications Inc. predicted that megawatt peak powers could be generated within months from XeF with about 1% efficiency and that krypton fluoride could reach up to 5% efficiency. Indeed, excimers took off in the months that followed, and our June 1976 issue reported that NRL had generated 130 mJ pulses from KrF in a modified commercial pulsed CO2 laser.
Megawatt military lasers
The 1970s saw US military researchers scale two gas lasers to impressive scales. The gas dynamic CO2 laser reached about 400 kW in the Air Force's Airborne Laser Laboratory, squeezed into a military version of a Boeing 707. The Navy built the mid-infrared advanced chemical laser, MIRACL, which reached two megawatts.
Both had serious problems, but that didn't deter Wyoming Senator Malcolm Wallop from proposing a fleet of 18 satellite battle stations, each armed with a 5 MW chemical laser and a 4 m mirror. He and four aerospace engineer advisors claimed the system could defend against "all Soviet heavy missiles, about 300 other intercontinental ballistic missiles, nearly all submarine-launched ballistic missiles, and all long-range bombers and cruise-missile carriers," we reported in our February 1980 issue. Our May 1981 issue reported DARPA would receive $69.1 million funding that year to develop the "Space Laser Triad," including a multi-megawatt hydrogen-fluoride laser called Alpha; $97.8 million was requested for 1982. In July 1981, we reported that the Airborne Laser Lab had failed to shoot down a test missile, but that did not deter the Reagan administration from including orbiting laser battle stations in the Strategic Defense Initiative two years later.
Our January 1987 market report put 1986 laser sales at $501.8 million, nearly half for gas lasers. CO2 sales reached $110 million, followed by ion with $85.6 million, He-Ne at $44 million, excimer at $19.3 million, and He-Cd at $6 million. Four gas lasers made our top-10 list of laser devices for 1986. PMS Electro-Optics (Boulder, CO) had two on the list: a 543 nm green He-Ne at 0.5 mW and a He-Ne tunable to lines at 632.8, 612, 604, and 594 nm. The other two were a battery-powered handheld 1 mW red He-Ne from Laseronix (San Marcos, CA) and a 6 in., 12 oz. red He-Ne laser pointer from Metrologic Instruments (Bellmawr, NJ).
Changes in the 1990s
Gas laser sales grew by the mid-1990s, but our January 1995 market report said their share of the overall $1.1 billion laser market in 1994 slipped to 37%. Excimer sales more than tripled to $61 million, fueled by uses in medicine and photolithography. However, He-Ne slipped to $38 million and was predicted to drop to $29 million in 1995, with unit sales decreasing from 309,000 to 186,000. A Toshiba ad on page 23 showed why He-Ne sales were slowing-diode lasers had reached 635 nm (see Fig. 4). I couldn't find a single ad or new product announcement for a He-Ne laser.In our February 1995 issue, Larry Marshall of Light Solutions (Acton, MA) wrote that the high prices of pump diodes might make it hard for solid-state lasers to challenge green He-Ne and argon-ion lasers "at least for the near future."
That took less than a decade. Our January 2006 market review showed ion laser sales—$121M in 1994—had dropped to $43M in 2005. Excimer sales more than made up for the difference, reaching $356M in 2005, dominated by $266M in sales for materials working, largely photolithography. CO2 sales, also mostly materials working, reached $680M in 2005. Both were rising fast.
Our April 2010 issue described the demise of the last megawatt-class gas laser weapon: the Airborne Laser. The chemical oxygen-iodine laser had run years behind schedule and billions of dollars over budget, and the Pentagon pulled the plug because it lacked the range to shoot down boost-phase missiles. Yet military researchers continue working on diode pumping of alkali vapor lasers, which could offer optical conversion efficiency of 90% or more and may be the best shot at megawatt-class gas lasers.
Today He-Ne and ion lasers are limited to uses requiring long coherence length and single-mode operation. However, CO2 and excimer lasers are alive and well because no other lasers can match their performance at wavelengths used for important applications.
Looking forward
New applications continue to emerge. Trumpf (Ditzingen, Germany) has developed a CO2 system with five amplifier stages delivering tens of thousands of pulses with peak powers of several megawatts to produce intense pulses of 13.5 nm extreme UV light for the next generation of semiconductor photolithography.
Research continues on new types of gas lasers, particularly outside of the bands well served by diode and solid-state types. One interesting area is development of gas lasers that operate inside hollow-core optical fibers. Researchers are taking confinement and energy transfer techniques developed for solid-state lasers and adapting them for gas lasers.
The concept grew from earlier studies of optical pumping and the use of gas-filled hollow fibers as optical frequency references, says Kristan Corwin of Kansas State University (Manhattan, KS). She, Brian Washburn of Kansas State, and Wolfgang Rudolph of the University of New Mexico (Albuquerque) led the team that demonstrated the first hollow-core fiber gas laser, exciting 3 μm lasing from acetylene (C2H2) with 1.5 μm pulses.1
"It's fun to have a new laser system to play around with," says Corwin. "Gain was so high in acetylene that we could observe lasing, despite the fiber's loss of 20 decibels/meter at 3 micron." Then Fetah Benabid, now at the Xlim Research Institute at the University of Limoges (France) developed the hypocycloid core fiber (see Fig. 5), which reduces loss at 3 μm by keeping most of the light in the gas.The hollow fiber geometry has important advantages. As in solid-core fibers, the long interaction length improves energy transfer in optical pumping, but the laser beam is guided in free space rather than in a solid. The gas core has a higher damage threshold, and offers the potential for flowing gas to improve heat flow.2 The group has recently demonstrated CW operation at 1280 to 1340 nm in a hollow-core fiber filled with molecular iodine and pumped at 532 nm. A recent paper in Optics Letters reports multiline output to 8 mW and several hours of laser operation without degradation.3
Discharge excitation is also possible. Having written his dissertation on discharge-pumped gas lasers, William Wadsworth of the University of Bath (UK) says, "I don't want it to go away." At Bath, he discovered that a hollow-core fiber could guide a free-space beam through cores down to 5 μm, and that got him thinking about gas discharge lasers.
Gain and output power in many conventional gas-discharge lasers increase as bore diameter decreases, but other optical considerations set a lower limit of about a millimeter. Hollow-core fibers offer tighter confinement. At CLEO 2014, Wadworth's group reported discharge excitation of a 3.5 μm He-Xe laser in a 1-m-long fiber with a 100 μm core, a step toward higher gain in the gas.4 He says getting the 20,000 V discharge down the meter-long fiber was easier than expected.
"We're still at the stage of showing this can be done, and then we will work out what we could use it for," says Wadsworth. Besides, he adds, "it's just fun," which is always a good way to learn.
REFERENCES
1. A. M. Jones, et al., "Mid-infrared gas filled photonic crystal fiber laser based on population inversion," Opt. Express 19, 2309–2316 (2011).
2. A. V. V. Nampoothiri, et al., "Hollow-core optical fiber gas lasers (HOFGLAS): A review [Invited]," Opt. Mater. Express 2, 948–961 (2012).
3. A. V. V. Nampoothiri et al., "Hollow core optically pumped I2 fiber gas laser," Opt. Lett. 40, 605–608 (2015).
4. S. A. Bateman et al., "Gain from helium-xenon discharges in hollow optical fibres at 3 to 3.5 μm" in CLEO: 2014 Postdeadline Paper Digest, OSA Technical Digest (online) (OSA, 2014), paper STh5C.10.
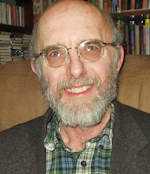
Jeff Hecht | Contributing Editor
Jeff Hecht is a regular contributing editor to Laser Focus World and has been covering the laser industry for 35 years. A prolific book author, Jeff's published works include “Understanding Fiber Optics,” “Understanding Lasers,” “The Laser Guidebook,” and “Beam Weapons: The Next Arms Race.” He also has written books on the histories of lasers and fiber optics, including “City of Light: The Story of Fiber Optics,” and “Beam: The Race to Make the Laser.” Find out more at jeffhecht.com.