Integrated Optics: Engineered lattice steers light around extremely tight corners
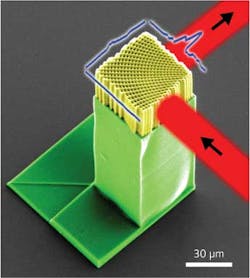
Researchers at the University of Central Florida (UCF; Orlando, FL) and the University of Texas at El Paso (UTEP) have shown that spatially variant photonic crystals (SVPCs) can be used to steer light beams efficiently around extremely tight corners, with potential applications in chip-to-chip optical interconnects.1 Other approaches—based on waveguides, metamaterials, surface plasmons, or other bulk-optic devices—require much larger bend radii or incur higher losses, and all require complex fabrication or alignment processes due to the subwavelength feature sizes and high-refractive-index or metallic materials involved.
While most chip-based optical devices steer light using refraction or waveguides, SVPCs steer light using diffraction and self-collimation. Self-collimation is a phenomenon where light propagates without spreading and is forced to follow an axis of the photonic-crystal lattice. Furthermore, SVPCs are composed of ordinary low-refractive-index dielectric materials with unit-cell dimensions on the order of 0.7λ (λ = wavelength)—much larger than the small dimensions required for metamaterials and other subwavelength bending techniques, making them easier and less expensive to fabricate.
The SVPC architecture is based on an algorithm developed by a UTEP team led by Raymond Rumpf that can be used to bend and twist lattices very abruptly without deforming the unit cells; such deformation would detune or destroy the self-collimation effect (see figure).
Fabricating SVPCs
The team at UCF led by Stephen Kuebler fabricated the SVPCs using multiphoton direct-laser writing (DLW), a form of 3D printing. A tightly focused pulsed laser beam is scanned within the volume of a photopolymer, like SU-8, to create multiple intersecting rods that cross to form a free-standing SVPC.
The present SVPC is based on a simple-cubic unit cell that forces light to propagate in the direction of the rods using the self-collimation effect. The refractive index of the material, the shape of the photo-patterned rods, and the fill factor of the unit cell were chosen so that the lattice can be manufactured by DLW and so that it exhibits self-collimation at a vacuum wavelength of 2.94 μm.
The UTEP algorithm stacks together multiple unit cells—adjusting their position, orientation, and form—to create an extended lattice with a 40 μm edge length that is smooth, continuous, free of defects, and keeps light collimated as it is propagates along a curved path through the SVPC. The geometry of the lattice produced by DLW was modeled more accurately by summing the rods and blurring the resulting pattern using a Gaussian function. The blur operation simulates acid diffusion that occurs during the DLW process in SU-8.
Controlling light
The UCF team experimentally characterized the SVPCs and showed that the peak-to-peak intensity ratio for bent and straight-through beams exceeds 8:1 for a lattice fill-factor of 44% to 52%, and drops to nearly zero for fill-factors significantly above 52%. Vertically polarized light is bent through the turn 25 times more effectively than horizontally polarized light because the rods have a prolate cross-section that introduces asymmetry into the unit cells of the SVPC lattice. These findings agree with electromagnetic simulations and show that SVPCs can be designed to control polarization and power flow through tight turns.
SU-8, like most photopolymers, is absorbing at wavelengths near 3 μm. The team is currently developing the next generation of SVPCs that function at shorter wavelengths, which are of greater interest for chip-to-chip communications and imaging. At these wavelengths, photopolymers and other materials have much lower loss.
“It appears we have achieved the tightest bend reported to date,” said Kuebler, “and we did so using lower refractive index materials than any of the other approaches. But this is only the beginning because SVPCs afford many other opportunities for controlling light.” The team is also exploring how SVPCs can be designed to control phase, improve coupling efficiency between waveguides, control propagation after exiting the lattice, and other parameters, which will greatly extend their utility in optical networks and imaging.
REFERENCE
1. J. Digaum et al., Opt. Express, 22, 21, 25788–25804 (2014).
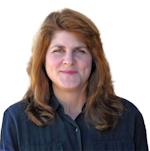
Gail Overton | Senior Editor (2004-2020)
Gail has more than 30 years of engineering, marketing, product management, and editorial experience in the photonics and optical communications industry. Before joining the staff at Laser Focus World in 2004, she held many product management and product marketing roles in the fiber-optics industry, most notably at Hughes (El Segundo, CA), GTE Labs (Waltham, MA), Corning (Corning, NY), Photon Kinetics (Beaverton, OR), and Newport Corporation (Irvine, CA). During her marketing career, Gail published articles in WDM Solutions and Sensors magazine and traveled internationally to conduct product and sales training. Gail received her BS degree in physics, with an emphasis in optics, from San Diego State University in San Diego, CA in May 1986.