Terahertz Sources: Terahertz QC metasurface VECSEL has near-Gaussian, low-divergence output
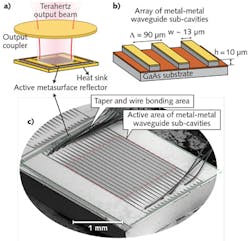
Since the demonstration of continuous-wave (CW) quantum-cascade (QC)-based terahertz lasers in the early 2000s, researchers have continued to increase power-output levels to the low milliwatt range, reduce divergence angles to around 10°, and improve beam quality.
Unfortunately, improving any one of these performance parameters is often at the expense of another, making simultaneous improvement of multiple parameters very challenging. For example, it is difficult to increase the output power for a semiconductor QC laser, as scaling up the waveguide-based gain cavity has the deleterious effect of increasing multiple-transverse-mode oscillations that lead to poor beam quality and modal instabilities. And because achieving terahertz wavelengths typically requires subwavelength metallic and/or plasmonic waveguide structures, the result is highly divergent beams with large side lobes unless special antenna-coupled distributed feedback (DFB) or photonic-crystal cavities are used.
An alternative design engineered by researchers at the University of California, Los Angeles (UCLA) to combat many of these performance tradeoffs is a vertical-external-cavity surface-emitting laser (VECSEL) operating at terahertz frequencies that consists of an amplifying metasurface reflector and a flat output coupler.1
The metasurface—a two-dimensional (2D) metamaterial surface—is essentially an array of antenna-coupled QC cavities, while the output coupler consists of an off-the-shelf wire-grid polarizer. The coupler provides a partially transmitting mirror to create the laser cavity and couple out a certain amount of power.
Beam engineering
The active metasurface reflector consists of metal-metal ridge waveguides of width w that sandwich the gallium arsenide (GaAs)/aluminum GaAs (AlGaAs) QC laser medium above and below the metal strips. Each of these 10-μm-thick sub-cavity waveguides are separated by the period Λ, which is less than the free-space wavelength λ0, to prevent diffractive losses or surface-mode excitation from the normally incident radiation.
These engineered subcavities confine the modes neatly to the active material within the waveguide on the GaAs substrate; however, they are designed with tapered absorbing regions at each end to suppress feedback and prevent lasing in the conventional confined waveguide modes. Rather, each subcavity is essentially an antenna that couples incident terahertz radiation to the QC-laser gain medium, amplifies it via stimulated emission, and reradiates it normal to the plane of the metasurface.
Taken collectively, an array of these subcavities acts as a "metasurface reflectarray" mirror that amplifies incident terahertz waves. Such an amplifying mirror can then be used to build an external cavity, which makes it straightforward to generate a high-quality beam. Because the metasurface response is polarization-specific, rotating the wire-grid polarizer used as an output coupler varies the transmission from the cavity for on-the-fly laser output coupling optimization.
Experimental results for 1.5 × 1.5 mm2 gain cavities (about 17 subcavity ridges) designed for nominal 2.9 THz operation confirm lasing from 2.8 to 2.9 THz under different bias conditions, and with ridge widths varying from 11.5 to 13.5 μm and a period of 90 μm. Of these variations, maximum lasing power occurred for a ridge width of 12.5 μm, which most likely has the best overlap of the gain spectrum with the metasurface resonance.
In an external-cavity configuration with a wire-grid polarizer coupler, the metasurface laser reaches 5.5 mW of output power with a 16.7 mW/A slope efficiency. The far-field beam pattern is near-Gaussian in shape with an approximately 5° divergence angle. Multiple methods for improving the CW laser output are envisioned for future research.
"This is the first time a reflectarray metasurface and a laser have been combined. This combination makes the VECSEL approach possible for terahertz wavelengths and provides a route to higher output powers simultaneously with excellent beam quality," says Benjamin Williams, associate professor of Electrical Engineering at UCLA. "The metasurface approach may in the future allow one to engineer the beam to have arbitrary polarization, shape, phase front, and spectral properties."
REFERENCE
1. L. Xu et al., Appl. Phys. Lett., 107, 22, 221105 (2015).
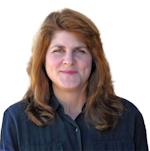
Gail Overton | Senior Editor (2004-2020)
Gail has more than 30 years of engineering, marketing, product management, and editorial experience in the photonics and optical communications industry. Before joining the staff at Laser Focus World in 2004, she held many product management and product marketing roles in the fiber-optics industry, most notably at Hughes (El Segundo, CA), GTE Labs (Waltham, MA), Corning (Corning, NY), Photon Kinetics (Beaverton, OR), and Newport Corporation (Irvine, CA). During her marketing career, Gail published articles in WDM Solutions and Sensors magazine and traveled internationally to conduct product and sales training. Gail received her BS degree in physics, with an emphasis in optics, from San Diego State University in San Diego, CA in May 1986.