Fiber Lasers: Unclad Yb:YAG crystalline fiber acts as purely waveguided laser
As is true with bulk solid-state lasers, transitioning from the use of doped glass to the use of a doped single-crystal gain medium is an important step in scaling up the output power of individual fiber lasers. For this reason, single-crystal fiber (SCF) is under intensive development. Various configurations have been created, including crystalline-clad crystalline fiber created using adhesive-free bonding (difficult to fabricate and thus not suitable for mass production), glass-clad crystalline fiber (not suitable for power scaling because of the thermal insulating properties of the glass cladding), and unclad SCF (which is very suitable for research, but will need a suitable cladding deposition technique for wider use).
Previous tested unclad SCF configurations have consisted of short (30–60 mm long) flood-illuminated crystalline rods with a 1-mm-or-less diameter in which the pump light bounces along inside the rod because of total internal reflection, but the mode structure of the output laser light itself is defined solely by the cavity mirrors—in other words, the SCF has not acted as a waveguide for the laser light and is more a bulk than a fiber laser.
In contrast, a longer and thinner diode-pumped unclad ytterbium YAG (Yb:YAG) crystalline fiber recently developed and tested by researchers at the U.S. Army Research Laboratory (ARL, Adelphi, MD) and Shasta Crystals (San Francisco, CA) has demonstrated pure waveguided laser operation (see figure).1 The SCF, which is 100 μm in diameter and 100 mm long with a 1% dopant concentration, was fabricated by Shasta Crystals using a laser-heated pedestal-growth (LHPG) technique potentially suitable for mass production of SCF (although it will require the development of a mass-producible crystalline cladding deposition method). The fiber enables experimentation and optimization of SCF.
High conversion efficiency
In the experimental setup, a non-antireflection-coated section of SCF is sandwiched between two laser cavity mirrors—light from a linearly polarized laser diode emitting at 969 nm with a 3 nm bandwidth is focused onto one end of the fiber, passing through the rear (dichroic) cavity mirror and into the fiber. The fiber sits loosely in a V-groove to confine it with minimal physical contact, while a convex lens collimates the laser's output. A converging lens, a laser-line filter, and a laser power meter allow collection of data on the laser output.
Since no thermal grease was used to draw heat from the fiber because of a resulting adverse effect on waveguiding, the diode pump was operated in a quasi-continuous-wave (QCW) mode at a 1% duty cycle to keep the fiber cool. The 1 ms pump pulse length is on the same order of the upper laser level lifetime of Yb3+ in YAG, and so the experimental results are representative of CW operation—just at a reduced thermal load.
Various output-mirror reflectivities from 18% to 70% were tried, with the optimum reflectivity found to be 25%. With a QCW pump input of 91.6 W (averaging out to a bit less than a watt because of the 1% duty cycle), the QCW laser output was about 53 W at a 1030 nm wavelength, showing that the fiber laser was efficient even in its unrefined experimental configuration.
As for the SCF parameters themselves, Giselle Maxwell, president and CEO of Shasta Crystals, explains that the 1% dopant level was determined by spectroscopy studies to help decide what concentration would provide the best conditions for lasing; the length of the crystal was determined by the same spectroscopy studies, which determine how long a crystal should be for a certain concentration of dopant to achieve maximum absorption of the pump beam and the most efficient lasing effect in the crystal; and the diameter of the crystal was determined as a function of convenience for the user and what effect was desired (smaller diameters lead to better waveguiding).
"Next steps are to improve on cladding technology (mostly thickness of the cladding) to provide a true all-crystalline double-clad single-crystal fiber that will beat the performance of any double-clad glass fiber because of improved thermal conductivity properties," notes Maxwell.
REFERENCE
1. J. Zhang et al., Laser Phys. Lett., 13, 075101 (2016); doi:10.1088/1612-2011/13/7/075101.
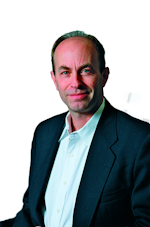
John Wallace | Senior Technical Editor (1998-2022)
John Wallace was with Laser Focus World for nearly 25 years, retiring in late June 2022. He obtained a bachelor's degree in mechanical engineering and physics at Rutgers University and a master's in optical engineering at the University of Rochester. Before becoming an editor, John worked as an engineer at RCA, Exxon, Eastman Kodak, and GCA Corporation.