IR Imaging: Spherical mirror enables internal stray-radiation measurements of cryogenic infrared imaging systems
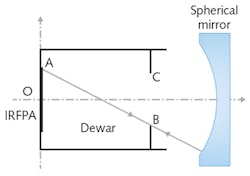
While stray light can be a problem for any type of optical system, it is an especially prominent concern for infrared (IR) thermal-imaging systems. This is the case for the simple reason that, in the thermal IR region, stray light is not just produced by objects outside the optical system—it is also produced within the system, which is radiating thermally.
One component in a high-performance thermal-imaging system that is often cryogenically cooled is the detector—for example, an infrared focal-plane array (IRFPA), which unavoidably potentially ends up being affected by the surrounding thermally radiating optical system. Thus, optical designers use various techniques to try to suppress internal stray radiation.
To understand whether these suppression techniques actually are doing their job or not requires a way to quantify the radiant flux produced within the system and the resulting effects on the system because of this internal stray radiation. One way to quantify this is through computer simulation—for example, using a ray-tracing or a Monte Carlo method. However, because no actual experimental measurements are taken in this approach, real-life results could be wildly different from the simulation and no one would know.
Experimental measurements are often taken on a finished system, but these measurement results typically contain elements of both internal and external stray radiation, and thus don't isolate the results of the internal stray radiation alone. Other experimental approaches are complex, involving, for example, bringing the entire system to two different temperatures and taking a set of measurements at each temperature, or partially disassembling the systems to take certain measurements.
Spherical mirror
Now, researchers from the Chinese Academy of Sciences (Changchun, China) and the University of Chinese Academy of Sciences (Beijing, China) have come up with an approach to experimentally measure internal stray radiation of cryogenic IR imaging systems using only a spherical mirror, with no temperature changes or partial disassembly required (although experimental measurements were taken at different ambient temperatures simply to fully characterize the approach).1
The function of the spherical mirror is to prevent stray thermal radiation from reaching the IRFPA. The mirror is positioned so that all rays traced from the IRFPA are reflected by the mirror back to the cryogenic dewar. The curvature of the spherical mirror and its positioning in relation to the dewar and IRFPA are laid out in a particular way to enable this to happen (see figure). To further facilitate optical isolation of the IRFPA, the high-reflection mirror coating is designed to have a very low emissivity.
For calibration, an extended-area blackbody reference source is placed just external to the optical system so that it covers the optics field of view, allowing effects of the atmosphere to be ignored. The system is then calibrated at several integration times.
To measure the internal stray radiation, the spherical mirror is placed in its position in front of the IRFPA and measurements over different integration times are taken. Calculations using all these measurements allow the system output and flux resulting from internal stray radiation to be determined.
Measurements on MWIR optical system
To verify their model, the researchers made measurements on a real midwave-infrared (MWIR) optical system with an aperture of 50 mm and a focal length of 100 mm. The system's cryogenic IRFPA had 640 × 512 pixels, operated over a 3.7–4.8 μm band, and an output noise of about 20 DN. The extended blackbody used in the experiment was 100 × 100 mm in size with an emissivity of 0.97 over the operating range of the IRFPA.
Data taken at various ambient temperatures ranging from 30° to 60°C showed that the measurements were linearly proportional to the integration time. Further measurements, with the blackbody at different temperatures than the optical system, correctly showed the nonlinear relation of internal stray radiation output to the ambient temperature (consistent with blackbody radiation theory).
The researchers add that the simplicity of the measurement technique means that other relevant information, such as changes because of structure distortion or the appearance of surface contamination, can be straightforwardly found by monitoring the internal stray radiation over time.
REFERENCE
1. Q. Tian et al., Appl. Opt. (2017); https://doi.org/10.1364/ao.56.004918.
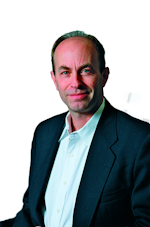
John Wallace | Senior Technical Editor (1998-2022)
John Wallace was with Laser Focus World for nearly 25 years, retiring in late June 2022. He obtained a bachelor's degree in mechanical engineering and physics at Rutgers University and a master's in optical engineering at the University of Rochester. Before becoming an editor, John worked as an engineer at RCA, Exxon, Eastman Kodak, and GCA Corporation.