Laser-induced Breakdown Spectroscopy: Compact industrial LIBS systems can assist aluminum recycling
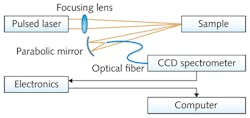
Laser-induced breakdown spectroscopy (LIBS) is an atomic-emission spectroscopy technique that enables rapid chemical analysis of a wide range of materials, including metals, semiconductors, glasses, biological tissues, plastics, soils, thin paint coatings, and electronic materials. The LIBS technology has received increased interest in recent years as a result of the development of more compact (including handheld) systems that enable in-field use and the construction of tools for on-line materials analysis. This development has been made possible by the increased availability of more compact and industrial-grade system components, including lasers and spectrometers.
A recent study conducted by the Swedish national research labs Acreo Swedish ICT and Swerea Kimab (both in Stockholm), in collaboration with laser manufacturer Cobolt AB, exemplifies this trend and shows how a new class of compact, industrial-grade lasers with multikilohertz pulse-repetition rates enables significant reduction of the footprint of a LIBS system and opens new opportunities for the deployment of LIBS in efficient metal sorting for recycling.
LIBS technique
The major strength of the LIBS technique is its ability to perform fast and remote chemical analysis to determine the elemental composition of samples under test without any sample preparation. The LIBS technology relies on focusing short, high-energy laser pulses onto the surface of a target sample to generate a plasma consisting of small amounts of ablated material (see Fig. 1).
The extremely high temperatures within the early plasma cause the ablated material to dissociate into excited atomic and ionic species; as the plasma cools, the characteristic atomic emission lines can be detected by a spectrograph. The method enables fast and sensitive chemical analysis of,cin principle, any kind of matter (solid, liquid, or gas).
Detection limits are typically in the low parts per million for heavy-metal elements. Sample preparation is normally unnecessary, and the method is also considered essentially nondestructive as such a small amount of the material is removed. Other advantages of LIBS are its ability to provide depth profiles and to remove surface contamination.
LIBS is an attractive technology for a wide range of scientific and industrial analytical applications, including metal-content analysis, solar silicon quality control, plant and soil analysis, mining and prospecting, forensic and biomedical studies, and explosives and biological warfare detection. Particularly interesting is its potential use in tools for on-line monitoring of industrial processes, especially for the metal industry. LIBS can, for example, be applied to monitor and optimize critical metallurgical processes (slag or molten metal analysis), to control the quality of metal products (rolls, tubes, foils, and so on), or to analyze and sort metal scrap before recycling.
Lasers for LIBS
Most laboratory LIBS set-ups have traditionally been based on flashlamp-pumped Q-switched Nd:YAG lasers that deliver pulses with energies of hundreds of millijoules in short pulse widths (4 to 5 ns) at relatively low pulse-repetition rates, typically 10 to 30 Hz. More recently, industrial fiber lasers have also been shown to provide good results in generating plasmas with millijoule pulses of slightly longer 40 ns pulse widths and with multikilohertz pulse rates.1 A major drawback of these laser sources, however, is their large size and high power consumption, which are strongly limiting factors for using LIBS in industrial and on-line applications.
Although high-pulse-energy lasers perform very well in many scientific LIBS applications, other types of laser sources that have a slightly different set of performance parameters and a much more compact formation can be considered for LIBS applications. The generation and properties of the plasma is affected not only by the pulse energy, but also by the laser pulse width, repetition rate, and wavelength.2-4 It is also clear that another important attribute of the laser is its beam quality, as this parameter affects the power density at the sample.
Improving efficiency in aluminum recycling
An example of an application that would strongly benefit from the availability of more compact and industrial-grade LIBS systems is aluminum recycling. Aluminum is in principle 100% recyclable; its recycling involves the collection of waste and subsequent use as secondary material in the production of new products. The use of recycled aluminum requires only 5% of the energy used in extraction of virgin minerals to produce aluminum, thus enabling large savings in energy consumption.A large part of the aluminum scrap on the market today is delivered from shredding mills, in which cars as well as industrial and household goods are cut into small pieces. The shredded material is inhomogeneous and, today, mostly sorted by visual inspection or coarse sorting techniques. The uncertainties in alloy composition of scrap materials set an upper bound to the amount of recycled aluminum used in production, where very stringent compositions are required.
Due to the lack of efficient aluminum scrap-sorting methodology, today only a limited fraction of recycled aluminum can be used in aluminum production. The potential for direct classification and sorting of recycled aluminum flows is therefore huge, both in terms of economic benefits to the aluminum producers and minimized environmental impacts.
Prototypes of LIBS systems have already been proposed and successfully demonstrated in the laboratory and at test sites for rapid classification of aluminum alloys, clearly showing the benefits of using LIBS to efficiently sort different alloys and dynamically handle and manage recycled material flows in scrapyards or production plants (see Fig. 2). Practical implementation and widespread use of LIBS as an on-line tool at scrapyards or at production sites requires, however, development of more robust, faster, and most of all more compact LIBS systems.
Experimental demo of more compact LIBS set-up
With the purpose of developing a LIBS system that could meet the requirements on robustness and compact size for use in industrial applications such as aluminum recycling, researchers at Acreo Swedish ICT and Swerea Kimab integrated a Cobolt Tor laser from Cobolt AB in their LIBS setup as an alternative to the high-pulse-energy, low-repetition-rate Nd:YAG laser previously used.A key advantage of the laser is its substantially more compact size compared to traditional high-pulse-energy Nd:YAG lasers. The laser head measures 125 × 70 × 45 mm and is accompanied by an electronics unit for supply of drive currents and control signals measuring 190 × 72 × 28 mm. Typical heat load of the laser head is less than 30 W, which, when combined with the small size, allows for compact integration into portable industrial LIBS systems. The laser is manufactured into hermetically sealed packages to ensure robust performance and long lifetime in varying ambient conditions such as those that exist for demanding industrial applications.
The LIBS set-up in this work involved a Cobolt Tor pulsed laser (1064 nm, 8 kHz, 4 ns, 150 μJ), a lens to focus the laser beam onto the sample to create a plasma, and collecting optics to transport the emitted plasma light to a compact spectrometer (the HR2000+ made by Ocean Optics, Dunedin, FL).A first round of experiments was conducted on aluminum reference samples to investigate the capability of the setup to classify different aluminum alloys with good confidence and also to compare the performance of this setup with spectra generated from a low-repetition-rate flashlamp-pumped Q-switched laser emitting pulses with energies of hundreds of millijoules. The two different laser types generate very similar spectra (see Fig. 5).
Encouraged by the promising results on reference samples, the research team proceeded to experiments with dirty scrap samples collected at scrapyards, aiming to confirm the practical applicability of a LIBS system based on compact high-repetition-rate lasers. The system was proven capable of clearly resolving the elemental composition of various alloys also from dirty scrap samples, as evidenced by the two spectra presented in Figure 6.The very strong performance of this compact high-repetition-rate laser in LIBS applications is most likely related to its very good beam quality, which enables high irradiance and fluence values. It is also believed that its relatively low-energy pulses create short-lived continuum plasma backgrounds, which allows the use of non-gated detectors also for quantitative analysis, greatly simplifying the detector requirements and system cost. The high repetition rate of the laser also contributes to enhancing the signal-to-noise ratio at the detector level.
Another advantage of this laser type is that its lower-energy pulses minimize the size of the ablation volume and therefore enable a nearly nondestructive analysis compatible with product quality control. Moreover, the high repetition rate and very low pulse-to-pulse jitter enable rapid scanning along a sample and allow for synchronized gating of the detection system, which could lead to higher signal-to-noise ratios and lower detection limits.
We conclude that the LIBS technology has great potential for use in tools for industrial analytical applications such as on-line scrap metal sorting for more efficient recycling. We have shown that the use of compact high-repetition-rate pulsed lasers with a high-quality beam can provide quality LIBS results while drastically reducing the system size, allowing for integration into portable LIBS systems suitable for use in industrial environments.
REFERENCES:
1. M. Scharun et al., Spectrochimica Acta Part B 87, 198 (2013).
2. L. Radziemski et al., Spectrochimica Acta Part B 87, 3 (2013).
3. R. Ahmed et al., J. Appl. Phys. 106 (3) (2009).
4. J.D. Winefordner et al., J. Analytical Atomic Spectrosc. 19, 1061 (2004).
5. B. Noharet et al., SPIE Photonics West, Vol. 8992 89920R-1 (2014).
Håkan Karlsson | CEO and Cofounder, Cobolt (HÜBNER Photonics)
Håkan Karlsson is CEO and cofounder of Cobolt, a part of HÜBNER Photonics (Solna, Sweden).
Bertrand Noharet | Group Manager, Acreo Swedish ICT
Bertrand Noharet is group manager at Acreo Swedish ICT (Kista, Sweden).
Tania Irebo | Senior Scientist, Swerea Kimab
Tania Irebo is senior scientist at Swerea Kimab (Kista, Sweden).