Novel Detectors: Squeezed-light gravity wave detector handles high power with low noise
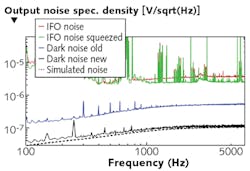
In late 2015, gravitational waves were detected for the first time using the powerful interferometers that comprise the Laser Interferometer Gravitational-wave Observatory (LIGO; http://ligo.org). Such a feat depends on extremely sensitive photodetectors that must be able to handle 5–50 mW of power on each photosensitive element while detecting noise levels as low as the quantum shot noise.
For these detectors to work well (to detect gravitational waves), the electronic noise of the detector (the photodiode electronics circuit) should be at least a factor of 10 less than the fundamental noise sources (like shot noise). This is even more difficult to accomplish when squeezed light is used—such as in the GEO 600 gravitational-wave detector—since the electronic noise is equivalent to optical loss and limits the squeezing factor, which likewise decreases the shot noise.
In the GEO 600, squeezed light is implemented by shooting a squeezed beam (precisely a squeezed vacuum state of light) into the nominal output port of the interferometer. This squeezed beam then replaces the vacuum fluctuation that would otherwise enter the interferometer. Vacuum fluctuations are the true cause of what is observed as shot noise upon detection of a light beam.
Characterizing shot noise as the "signal" noise in the squeezed-light detector, researchers from the Max-Planck-Institut für Gravitationsphysik (Albert-Einstein-Institut) and Leibniz Universität Hannover (both in Hannover, Germany), the California Institute of Technology (Caltech; Pasadena, CA), and SUPA, the School of Physics and Astronomy at the University of Glasgow (Glasgow, Scotland) have designed and implemented a new electronic photodetection circuit design that reduces electronic noise in the audio band, improving the shot-noise-to-electronic-noise ratio of the GEO 600 detector by a factor of more than 4 above 1 kHz and improving dynamic range by a factor of 7. With a maximum detectable photocurrent of 20 mA, the new circuit increases the observed squeezing level in GEO 600 by 0.2 dB.1
Removing existing limitations
Photodiode circuits can convert light energy (photocurrent) to voltage using ohmic resistors. Mathematical calculations of signal-to-noise ratio (SNR) reveal that two major limitations to achieving high SNR are the voltage across the resistor and the resistor temperature. However, reducing these parameters requires impractical cooling systems to remove resistive heating.
To date, gravitational-wave detectors achieve SNR values between 6 and 9. When using squeezed light, it becomes even more difficult to achieve SNR values greater than 10 because squeezed light reduces the shot-noise level. Rather than using high voltage and resistive cooling, the researchers instead considered the effect of frequency in the current-to-voltage conversion process and extended the resistive circuit with an induction circuit.
The induction circuit allows the low-frequency (DC) component of the photocurrent to bypass the conversion resistor, which allows the use of larger resistor values. Since the SNR is limited by the Johnson thermal noise of the resistors, a larger resistor value will increase the SNR. While this principle has been used for radio-frequency applications, this is the first implementation of this kind in the audio-frequency band.
Using the original GEO 600 circuit design, which is also used by all other gravitational-wave detectors, a SNR of 7 was achieved in practice and a theoretical SNR of 10 was possible. But using the new induction circuit, SNR increased to 30—and more than 20 with squeezed light (see figure). Furthermore, the observed light squeezing improved by 2.5% or 0.2 dB, making it possible to detect more gravitational waves.
"Squeezed light will be implemented in gravitational-wave detectors other than GEO in the future," said LIGO scientist Hartmut Grote. "To unlock the full potential of squeezing, it is essential to detect the remaining shot noise with very high SNR. Our new circuit design makes this possible. The improvement may look small, but it is worthwhile pursuing to make laser interferometers such as LIGO, that have cost hundreds of millions of dollars, ever more sensitive."
REFERENCE
1. H. Grote et al., Opt. Express, 24, 18, 20107–20118 (2016).
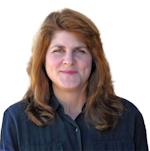
Gail Overton | Senior Editor (2004-2020)
Gail has more than 30 years of engineering, marketing, product management, and editorial experience in the photonics and optical communications industry. Before joining the staff at Laser Focus World in 2004, she held many product management and product marketing roles in the fiber-optics industry, most notably at Hughes (El Segundo, CA), GTE Labs (Waltham, MA), Corning (Corning, NY), Photon Kinetics (Beaverton, OR), and Newport Corporation (Irvine, CA). During her marketing career, Gail published articles in WDM Solutions and Sensors magazine and traveled internationally to conduct product and sales training. Gail received her BS degree in physics, with an emphasis in optics, from San Diego State University in San Diego, CA in May 1986.