Free-space Optical Communications: Building a 'deeper' understanding of underwater optical communications
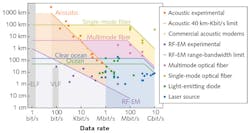
JOHN MUTH
The underwater environment is challenging for all modes of communications, with distinct tradeoffs between range and data rate. Radio waves are exponentially attenuated by the high electrical conductivity of seawater. Acoustics provide a long operational range (kilometers) at low data rates (kilobits/second), but typically have poor performance in shallow waters because of ocean noise and multiple acoustic reflections from the surface and bottom. This creates intersymbol interference (signal distortion).
Underwater free-space optical (FSO) communications using blue or blue-green lasers and light-emitting diodes (LEDs) provides niche, short-range (<150 m), high-bandwidth (megabits/second) solutions with ranges that are highly dependent on water quality. At North Carolina State University (NCSU), we focus on the practical considerations of building and implementing optical communication systems for underwater vehicles and robots, as well as underwater construction and subsea factories.
Data rate vs. range
The bandwidth requirements for underwater operations can range from about 1 kHz for control signals to telemetry that may require 10 times as much bandwidth to transmit data or imagery. To provide a frame of reference, telephone-quality audio generally requires approximately 3 kHz of bandwidth, while high-quality streaming compressed video needs around 500 Kbit/s.
In general, an optimized radio frequency (RF) communication system approaches about 1 bit/Hz of available bandwidth, suggesting the use of high carrier frequencies. However, the penetration of radio waves into water strongly depends on the conductivity and frequency, and traditionally has limited communication with submarines to very low frequency (VLF) and data rates on the order of 100 bits/s. Recently, for short distances of a few meters, some modems can transmit at kilobit rates.
The traditional mode of communications between underwater vehicles has been acoustic. The velocity and attenuation of sound in water is dependent on frequency, with higher frequencies being strongly attenuated and intersymbol interference limiting commercial systems to <500 kbit/s.
Underwater video is becoming increasingly important and is very challenging for acoustics, even when frame rates are reduced and compression algorithms are used. Optical wireless, on the other hand, can easily transmit at 1 to 5 Mbit/s using light-emitting diodes (LEDs) and at gigabit data rates using diode lasers (see Fig. 1). There have been recent efforts to increase the bandwidth of LEDs that may open additional opportunities for higher-data-rate, LED-based communications.
Going deep with optics
Structures permanently placed on the seabed can be serviced by underwater fiber-optic cables that deliver very high bandwidth communications at gigabit rates between subsea nodes. However, compared to guided modes in optical fibers, the underwater FSO communications proposition is more complicated.
Terrestrial FSO systems have bulk optics to minimize beam divergence, good pointing and tracking systems, and multi-wavelength adaptive optics to handle atmospheric turbulence. Such systems can currently provide gigabit data rates over kilometer-length scales. These capabilities have been greatly accelerated by leveraging eye-safe 1.55 μm lasers, erbium fiber amplifiers, and detectors developed for fiber-optic communications.
Because near-infrared (near-IR) wavelengths are strongly absorbed by water, a challenge for underwater communications has been finding bright blue-green lasers and LEDs. Fortunately, high-brightness gallium-nitride (GaN)-based LEDs and diode lasers now provide inexpensive light sources for small underwater platforms, while frequency-doubled near-IR lasers provide high peak powers for larger pulsed systems.
A second challenge is that during daylight hours for shallow depths, the sun is a very bright interfering light source that limits underwater detector performance, so there is a need for good optical filtering and wide dynamic range detectors.
Finally, compared to terrestrial FSO communications, turbulence is not the dominating factor in undersea communications systems. Instead, absorption and scattering are the major issues to be addressed. In clear-blue ocean water, maximum transmission is achieved at blue wavelengths (405–440 nm), with the absorption coefficient approximately 0.017 m-1. In coastal waters, the absorption coefficient is about twice as much-approximately 0.033 m-1, with the transmission maximum shifting to the green (510–530 nm). In the most turbid, yellow-matter-rich coastal water, the absorption is approximately 0.291 m-1 with the minimum absorption in the yellow.
Based on these values, the expected transmission range for optical wireless communications in the ocean is 150–200 m in very clear ocean waters, 50–75 m in ordinary ocean waters, and only a few meters in turbid harbor water. Unlike acoustics, the multipath dispersion from scattering is very small and is almost negligible until operating at more than 1 Gbit/s data rates.
Underwater system design
Communication system designers are interested in the ranges at which a system can perform, as well as the size of lenses needed and the field of view and pointing accuracy required to complete the link between transmitter and receiver. Underwater, these relationships are not always intuitive.
Unlike the strongly forward-scattered light in very clear water, in turbid water the short mean free path greatly expands the beam, allowing systems with wide-field-of-view optics to efficiently capture the photons that diffuse toward the receiver. These situations can be modeled using Monte Carlo simulations and compared with the experiment (see Fig. 2). In turbid water, the pointing requirements of the system can be decreased.Similarly, multipath dispersion—determined by increased path length as the phonons scatter between particles—can be computed and compared under different transmitter-receiver geometries as a function of water quality. It turns out that for the relatively short ranges expected for underwater optical communications at megabit rates, multipath dispersion is almost negligible.1
At NCSU, we have found that optical wireless systems can be very compact and that error correction coding and adapting the transmission rate as a function of range can improve signal-to-noise by 6–8 dB (see Fig. 3).2 While the increased signal-to-noise translates into increased range, error correction increases the robustness of the link.Like optical fiber communications, wavelength-division multiplexing using different-colored LEDs also allows the bandwidth to grow proportionally with the number of wavelengths. Smart transmitters and receivers that emit beams transmitting different codes, pointing in different directions, allows Code Division Multiple Access (CDMA) techniques similar to cell phone communications.3 We have also demonstrated a microelectromechanical (MEMS)-based retroreflecting modulator with sufficient bandwidth for video rates and software-defined radio using quadrature amplitude modulation (QAM).4,5
Most optical wireless links have been experimental efforts taking place in tanks or pools. Commercially, Ambalux (Tucson, AZ) demonstrated a 10 Mbit/s system with a range of 40 m and lists options for their optical transceivers at 100 Mbit/s and 1 Gbit/s transmission rates. Technology developed by the Woods Hole Oceanographic Institute (Woods Hole, MA) has transitioned to Sonardyne (Yateley, England), which now lists two BlueComm [trademarked] systems using LEDs for 10 m at 1–5 Mbit/s, 150 m at 1–12.5 Mbit/s, and a 7 m laser-based system at 500 Mbit/s.
In the past year, several researchers have also performed tank experiments that start to push the limits of optical wireless communications in the laboratory using 16 QAM orthogonal frequency division multiplexing (OFDM) to achieve data rates in excess of 7 Gbit/s in seawater for ranges of 4 to 8 m.6,7 This suggests that optical wireless communication technology is rapidly maturing and that experiments need to move from tanks in laboratories and out to sea.
In general, as humans begin to more efficiently exploit undersea resources, an increased network of undersea pipelines, vehicles, divers, and robots will need to operate together to inspect, repair, and maintain underwater equipment. Multiple modes of communications will be required and hybrid systems that seamlessly convert between acoustic, fiber-optic, RF, and underwater FSO communications will become increasingly important.
In this emerging undersea world, wired communication and power cables will connect underwater fixed infrastructure and tethered vehicles, sonar and RF will serve subsea platform-to-shore communications, and underwater FSO architectures will provide a vital, niche communications role by offering high-bandwidth, short-range communications between fixed and moving assets like underwater autonomous remotely operated vehicles (ROVs) and divers.
REFERENCES
1. B. Cochenour and L. Mullen, "Channel response measurements for diffuse non-line-of-sight (NLOS) optical communication links underwater," Proc. IEEE/MTS OCEANS, Waikoloa, HI (Sep. 2011).
2. J. A. Simpson et al., "5 Mbps optical wireless communication with error correction coding for underwater sensor nodes," Proc. IEEE/MTS OCEANS, Seattle, WA (Sep. 2010).
3. J. A. Simpson et al., IEEE J. Sel. Area. Comm., 30, 5, 964–974 (Jun. 2012).
4. W. C. Cox et al., "A MEMS blue/green retroreflecting modulator for underwater optical communications," Proc. IEEE/MTS OCEANS, Seattle, WA (Sep. 2010).
5. W. C. Cox et al., "Underwater optical communication using software defined radio over LED and laser based links," Proc. IEEE Military Communications Conference (MILCOM), Baltimore, MD, 2057–2062 (Nov. 2011).
6. H. H. Lu et al., IEEE Photon., 8, 5, 7906107 (Oct. 2016).
7. T. C. Wu et al., Sci. Rep., 7, 40480 (Jan. 2017).
John Muth is a professor in Electrical and Computer Engineering at North Carolina State University, Raleigh, NC; e-mail: [email protected]; www.ece.ncsu.edu.