Photonics Products: Molded Optics: Precision glass molding boosts gamut of optics
The fabrication of a modern precision optical component from a hunk of glass or other material is a marvel of engineering, considering that the finished component (or assembly of components), when in use, usually is required to introduce a maximum wavefront error of, say, less than a wave (λ), and often much less than that (sometimes down to λ/10 or better). In addition, the surface must be virtually featureless at microscopic and nanoscopic dimensions so as not to scatter too much light.
These two requirements for a precision optical component—having the correct surface shape at scales much larger than the wavelength of light (to achieve the intended wavefront shape), and having minimal smaller features (to achieve low scatter)—must happen together, of course. Fortunately, the modern optics industry has a number of effective fabrication approaches under its belt, each with its own advantages.
The baseline approach, of course, is grinding and polishing and its modern variants, such as magnetorheological finishing (MRF). A second technique, which allows the deterministic precision shaping of optical components made from a restricted set of materials (for example, metals, some semiconductors, and polymers), is single-point diamond turning. And a third, which has the two advantages of straightforward mass-production capabilities, and production of arbitrary surface shapes with minimal extra effort, is molding. (Note that these techniques can be used to produce both reflective and refractive optics.)
Here, we will be discussing molded optics. Because there are so many types of molded optics—glass optics, plastic (polymer) optics, polymer on glass or metal, molded lens or mirror arrays, and spheres, aspheres and freeform shapes, low-precision and high-precision—we will narrow the field down to precision glass molded refractive optics, as well as a closely related technique (a thin polymer aspheric layer added to a glass lens).
Precision glass molding of chalcogenide glass
Precision glass molding (PGM) is a high-temperature compression-molding process that can be used to manufacture optical elements in high volume, explains Alan Symmons, executive vice president of operations at LightPath Technologies (Orlando, FL). "PGM is most commonly used to mold aspheric lenses, but is also used to mold arrays, aspheric-cylindrical lenses, V-grooves, rod lenses, and other optical elements," he says. "There are over two hundred moldable glass types to choose from, with transmission ranging from the visible to the longwave-infrared (LWIR)."
For example, precision glass molding of chalcogenide glass (ChG), combined with the recent reduction in the cost of microbolometers, has enabled a new generation of low-cost thermal imaging devices, Symmons notes. Consumer-based handheld thermal imagers, commercial thermal weapon sights for hunters, and thermal camera add-ons for cell phones have all become readily available to the average consumer in the last few years because of this technology.
"Chalcogenide glass enabled these advances because it is an amorphous material that can be molded, unlike its more traditional competitors: germanium (Ge), zinc sulfide, zinc selenide, and so on," Symmons says. "Chalcogenide glasses such as LightPath's BD6 (As40Se60) provide a much lower-cost material that can be manufactured using PGM, a more suitable process for high-volume manufacturing required for consumer products."
LightPath's ChGs also provide technical advantages over Ge, according to Symmons (see Fig. 1). When compared to Ge, ChGs are lighter in weight, have improved transmission at elevated temperatures (Ge is opaque at temperatures higher than 65°C, while ChGs continue to transmit), and are inherently athermal—an important consideration in thermal imaging.
It should be noted that Symmons is the coauthor, along with Michael Schaub, of a book on the topic of molded optics, both plastic and precision glass. Called the Field Guide to Molded Optics, the book was published in 2016 by SPIE Books (doi:10.1117/3.2230581; eISBN: 9781510601253; print ISBN13: 9781510601246).
Using advanced metrology
Precision glass molding is also known as "isothermal glass molding," and has a longer process time, and therefore higher costs, compared to nonisothermal molding, says Andreas Kunz, business director, advanced optical components at Fisba (St. Gallen, Switzerland). (An isothermal process is one that happens at a constant temperature.) However, Kunz notes, the higher level of precision attained by this method outweighs the extra cost, and enables solutions for applications in high-performance imaging systems and in the field of laser-beam shaping.
"In terms of materials, Fisba's capabilities range from oxide glass for applications in the visible and near-IR spectrum to chalcogenide glass for the shortwave-IR (SWIR), midwave-IR (MWIR), and LWIR ranges," Kunz says. "Precision molded components include aspherical lenses, off-axis lenses, cylindrical lenses, off-axis cylinders, and cylinder arrays. In the case of chalcogenide glass lenses, diffractive structures can also be molded directly onto the lens surface. The latest progress in the field of oxide glass is the molding process for freeform components."
Fisba works with both batch- and transfer-type molding machines. Kunz explains that a key success factor is advanced metrology, which includes the use of a Panasonic UA3P tactile-measurement instrument for aspheres, freeforms, and arrays. Complementary operations like center grinding, truncating, coating, and cementing of components are also done by Fisba.
For many years, Fisba has been molding acylindrical (noncircular cylindrical) single components and arrays that are used mainly as micro-optics for diode-laser collimation. "The latest innovation is a freeform slow-axis collimator (SAC) lens that helps to improve the beam quality in both the slow axis and fast axis," Kunz says (see Fig. 2). "In testing, a demonstrator component was used in a diode-laser system at a blue (450 nm) wavelength. However, the concept can be transferred to near-IR and similar improvements can be expected. The improvements, measured as the relative increase of the enclosed energy, are as high as 40% at 450 nm."
Fisba's molding technology allows for the production of several SAC lenses on one wafer, Kunz notes. These lenses are then diced by a wafer saw. "This example shows how a process chain consisting of various complex steps, in combination, lead to innovative products," he says. "Optical design, metrology (optical and tactile), tooling, molding, coating, and grinding need to be controlled with high precision, whereas knowledge about the molding process is important for achieving a good design from both a serial production and application standpoint."
Polymer asphere surface on glass
In addition to injection-molded acrylic aspheres, Edmund Optics (Barrington, NJ) produces precision molded aspheres made from D-ZK3 glass, as well as polymer-molded aspherized achromats consisting of a spherical glass achromat and an aspheric polymer surface, says Cory Boone, an optical engineer at Edmund.
"Aspherized achromats are cost-effective lenses that achieve excellent correction for both chromatic and spherical aberrations by combining a glass achromatic lens with a photosensitive polymer using a unique polymer molding process," Boone says. "The polymer is applied only on one face of the doublet through an easily repeatable procedure (see Fig. 3)."
Boone explains that the polymer surface reduces wavefront errors present in typical achromats while boosting numerical aperture. "They have better color correction than standard achromatic lenses, and they have similar spherical aberration correction to machined aspheres," he says. "This results in an economical means of meeting the stringent demands of today's optical and visual systems."
By incorporating aspherized achromatic lenses into lens systems, great improvements in chromatic and spherical aberration can be made in applications including fiber-optic focusing or collimation, image relay, inspection, beam expanders, scanning, and high-numerical-aperture imaging systems, Boone adds. For example, in a 1:1 relay pair using two standard achromatic lenses, one or both of the achromatic lenses could be replaced by an aspherized achromat to greatly improve spherical aberration correction for little extra cost, resulting in a higher-resolution image.
Specialized shapes
Precision glass molding of optical components is not limited to production of single lenses. A wide range of more-specialized shapes, including lens arrays and more, are available (see Fig. 4), most of which would be difficult to make economically any other way (although embossing, a technique in which a metal mold is pressed into softened glass, is another mass-production approach being developed, for example, to create microlens arrays for illumination uniformizers).
For More Information
Companies mentioned in this article include:
Edmund Optics
Barrington, NJ
www.edmundoptics.com
Fisba
St. Gallen, Switzerland
www.fisba.com
Isuzu Glass
Torrance, CA
www.isuzuglass.com
LightPath Technologies
Orlando, FL
www.lightpath.com
DISCLAIMER: While we try to include information from the broadest possible number of companies that manufacture the products featured in our Photonics Products series, because of limited word count as well as deadlines that cannot always be met by requested contributors, we cannot possibly include all companies and regret if your company is not included in our series.
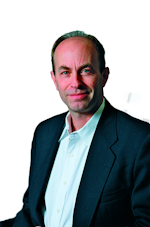
John Wallace | Senior Technical Editor (1998-2022)
John Wallace was with Laser Focus World for nearly 25 years, retiring in late June 2022. He obtained a bachelor's degree in mechanical engineering and physics at Rutgers University and a master's in optical engineering at the University of Rochester. Before becoming an editor, John worked as an engineer at RCA, Exxon, Eastman Kodak, and GCA Corporation.