Quantum Technology: Quantum sensing is gaining (s)pace
When Peter Leibinger, vice chairman and CTO of Trumpf (Ditzingen, Germany), gave the opening plenary speech at the 2016 LASER World of PHOTONICS trade fair in Munich, he highlighted quantum technology as the next big thing in photonics. The trend is well recognized by politicians and investors as well: Governments and industry giants from China, the U.S., Canada, and Europe are currently aligning their funding programs to catch up in the race for quantum technology leadership.
Funding: Billions to be spent
In March 2017, The Economist published an impressive review of the state of quantum research. Included were numbers for quantum technology (QT) engagement: About 7000 people with a combined budget of €1.5 billion were working on QT in 2015 (numbers from McKinsey). A subsequent summary from the European Commission estimated that budget to grow to €5 billion within five years.
In 2016, the European Commission initiated the Future and Emerging Technologies (FET) flagship project for quantum technologies. Such a 10-year, €1 billion project was first started in 2013 for graphene, and the second was the Human Brain Project. The Quantum Technologies Flagship and a quantum manifesto call for funding applications that are expected in the first quarter of 2018. This project comes along with approximately two dozen national initiatives.
China has made the most progress in quantum technology recently. With the Micius project, they successfully showed satellite-to-ground quantum communication. Although that hit the global community like a small Sputnik shock, it is just one result of their numerous activities.
In summer 2017, the Chinese Academy of Sciences inaugurated its own Institute of Quantum Information and Quantum Technology Innovation in Anhui, China. A review of the Chinese activities from the American Jamestown Foundation claimed "virtually unlimited funding" for QT projects in China. This report also lists numerous activities in the field of quantum sensing going on for more than a decade, particularly in quantum radar and quantum navigation.
It does not include the recent activities of China’s largest private investor: In October 2017, the Alibaba group announced their plans to invest more than $15 billion over three years in researching emerging technologies including artificial intelligence and quantum computing. They plan to open seven research labs in China, the U.S., Russia, Israel, and Singapore.
For a long time, the U.S. was on the forefront of QT research efforts. But this lead can be strongly questioned by the current developments in China. In summer 2017, the U.S. National Photonics Initiative released a whitepaper calling for a National Quantum Initiative and $500 million funding. This led to a hearing in the U.S. House of Representatives titled American Leadership in Quantum Technology on October 24th. The hearing also included representatives from IBM, U.S. government agencies, and academia. Further progress remains to be seen.
Quantum sensing is already there
While the discussion of odd quantum phenomena such as Schrödinger’s famous cat (which is simultaneously alive and dead) is about a hundred years old, the industrial exploitation has yet to be shown. With such a second quantum revolution (taking the discovery of quantum physics as the first), several technologies are under discussion: Quantum cryptography and quantum computing certainly attract the most curiosity, but despite some successful proof of principle experiments, they are still very limited in their application.
Quantum enhanced imaging and quantum sensing are not as popular, but might be closer to practical realization. Both offer maximum precision at the fundamental limits. Quantum imaging is being investigated for military purposes (ghost imaging), for nanolithography, and for new medical diagnostics (Q-optical coherence tomography).
Quantum sensors take advantage of the quantum nature of matter to measure physical quantities such as frequency, acceleration, rotation rates, electric and magnetic fields, or temperature with the highest relative and absolute accuracy. These measurements allow for local and reproducible calibration with direct relation to natural or fundamental constants.
This is relevant for basic measurements of time or gravity—with the highest accuracy. The first is necessary for navigation systems, the second may allow for inertial navigation where precise acceleration measurement is used to achieve position data. Or it may support geological applications, including detailed mapping of hidden natural resources such as oil or water.
Measuring time in unprecedented precision was one of the first widespread applications of quantum technology. Now it is making its way to Earth’s orbit, where substantial economic benefits are expected: A new generation of navigation or telecommunication satellites would strongly benefit from atomic clocks.
What it takes to measure quantum effects
In its core, quantum sensors exploit the quantum mechanical behavior of atoms or ions. Lasers are the typical tools to prepare the special quantum states of the respective specimen. Typically, continuous-wave lasers are used to cool down atoms until their quantum properties dominate thermal effects. Then, laser pulses can be used to test spectroscopic properties of the specimen.
In an atom interferometer, for example, the wave functions of cold atoms can interfere quite similarly to optical waves as we know from optical slit experiments. Different from photons, atoms have a finite mass and so their interference pattern depends on gravity. Hence, the interference pattern can be used for ultraprecise gravity measurements.
From a physical point of view, such a sensor is simple and delivers reproducible results. Mostly, it doesn’t need calibration and it doesn’t drift—it’s just about counting frequencies. And measuring frequencies opens the door to the most precise measurements we know.
The lasers used in such sensor systems must be very specific, sometimes with quite extreme requirements. Typical laser linewidth requirements range from the megahertz to the sub-hertz level. For some applications, spectral purity is critical—that is, optical power contained in laser side modes or (amplified) spontaneous emission are detrimental to the performance of the sensor.
Lasers for quantum sensing space missions
A team at the Ferdinand-Braun-Institut in Berlin (FBH) developed a number of such lasers, particularly for space-based sensors, which makes the challenge even more difficult. Their partners at the Humboldt-Universität zu Berlin (HUB) took the lead for laser system integration. The Berlin activities were part of the German QUANTUS consortium led by the University of Hannover, a project dedicated to the investigation of cold atom effects under micro-gravitation.
Obviously, laser systems for space applications must be small and stable with long lifetimes. The workhorse that the FBH team developed is a micro-integrated diode laser. A typical version delivers more than 3 W at 780 nm out of a 40 g module. Shock testing went up to 1.500 g and the lifetime can be up to 100,000 hours mean-time-to-failure (MTTF).
In January 2017, an experimental setup was launched on board a sounding rocket from Kiruna, in northern Sweden, and demonstrated the first Bose-Einstein-Condensate (BEC) in space. The experiment used a diode laser system optimized for laser cooling and atom interferometry with ultra-cold rubidium atoms. Sounding rockets such as the one for this Matter-wave Interferometry in Microgravity (MAIUS) mission reach an apogee of about 250 km and offer about 6 min. of experimental time in micro-gravity.1
When a BEC was first experimentally shown in 1995, the setup filled an optical laboratory. For the MAIUS mission, the laser system was reduced to a volume of 21 liters and a mass of 27 kg. The total setup, including batterie s, electronics, lasers, and physics package, was integrated in a cylinder with 500 mm diameter and 2.79 m length (see Fig. 1). For this mission, the FBH team developed hybrid micro-integrated semiconductor laser modules that are suitable for application in space.
The whole system used four master-oscillator-power-amplifier (MOPA) laser modules. The master oscillator is a distributed feedback diode laser (DFB) with a linewidth of approximately 1 MHz followed by a tapered amplifier (PA) generating an output power of more than 1 W. An additional laser module is frequency-stabilized to a suitable transition of the rubidium atoms contained in a gas cell. It serves as a frequency reference for the MOPA laser modules that interrogate the rubidium atoms trapped in the physics package. The laser output of all MOPA modules is transmitted to a special Zerodur-based switching and distribution module, where the different laser beams for cooling and trapping are formed.
These laser modules, together with the Zerodur-based optical benches that were provided by partners of the consortium, have been integrated into one housing (see Fig. 2) and qualified by the HUB team to provide the laser subsystem of the scientific payload. After the successful 2017 flight, the system reached the ultimate technology readiness level 9 for sounding rockets.The next mission has been scheduled: the JOKARUS (German: Jod Kamm Resonator unter Schwerelosigkeit) mission will launch a payload on a sounding rocket and test an iodine frequency reference. This will be the first absolute optical frequency reference at 1064 nm relevant for a number of future missions in the context of global satellite navigation systems (GNSS), gravitational detection (LISA), or measurements of Earth’s gravity (NGGM).2
The JOKARUS mission is scheduled for spring 2018. The frequency reference is based on modulation transfer spectroscopy of a hyperfine transition in molecular iodine at 532 nm, using a frequency-doubled extended cavity diode laser (ECDL). The laser module delivers 570 mW at 1064 nm through a single-mode polarization-maintaining fiber, with spectral width of only 26 kHz (see Fig. 3). Shock tests at 8 gRMS have been successfully completed.What’s next?
The development of optomechanical setups for quantum sensors that are miniaturized, robust, and field- and space-deployable is the medium-term target of the Berlin-based teams. With a modular construction, it should be easy to realize different experimental functions such as atom interferometry or optical clocks. Demand and funding are expected from space agencies and telecommunication or navigation enterprises.
To foster local activities, the Ferdinand-Braun-Institut and the Humboldt-Universität zu Berlin joined forces already in 2008, when the Joint Lab for Laser Metrology was founded at FBH. The idea behind this Joint Lab concept is to bring together quantum physicists that provide deep insight into the laser requirements of quantum sensors and diode laser experts who know how to tailor diode lasers and micro-integration to specific needs.3
The roadmap toward this target includes further research and development on materials and assemblies with a particular focus on electro-optical technology, including integration methods and, of course, further testing.
The perspectives for the long term are even more interesting, as miniaturized and sealed systems can be applied on Earth as well as in space. But down here, there are many more applications—oil, gas, or water exploration is one thing, while a detailed mapping of the ground for buildings could be an even bigger market.
Such quantum sensing applications have a potential as big as pure IT applications of quantum technologies, and they might come much earlier.
REFERENCES
1. V. Schkolnik et al., Appl. Phys. B, 122, 217 (2016); doi:10.1007/s00340-016-6490-0.
2. V. Schkolnik et al., EPJ Quantum Technol., 4, 9 (2017), doi:10.1140/epjqt/s40507-017-0063-y.
3. A. Wicht et al., "Narrow linewidth diode laser modules for quantum optical sensor applications in the field and in space," Proc. SPIE, 10085, 100850F (2017); doi:10.1117/12.2253655.
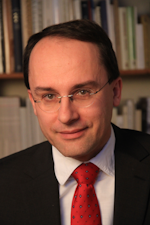
Andreas Thoss | Contributing Editor, Germany
Andreas Thoss is the Managing Director of THOSS Media (Berlin) and has many years of experience in photonics-related research, publishing, marketing, and public relations. He worked with John Wiley & Sons until 2010, when he founded THOSS Media. In 2012, he founded the scientific journal Advanced Optical Technologies. His university research focused on ultrashort and ultra-intense laser pulses, and he holds several patents.
Andreas Wicht | Head, Joint Lab Laser Metrology at Ferdinand-Braun-Institut
Andreas Wicht heads the Joint Lab Laser Metrology at the Ferdinand-Braun-Institut, Leibniz-Institut für Höchstfrequenztechnik (Berlin, Germany).
Markus Krutzik | Group Leader, Optical Metrology Group at Humboldt-Universität zu Berlin
Markus Krutzik is a group leader of the Optical Metrology Group at Humboldt-Universität zu Berlin (Berlin, Germany).