Nonlinear Optics: Femtosecond lasers and nonlinear optics: New approaches solve old problems in ophthalmology
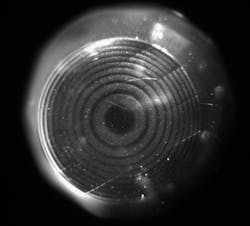
WAYNE H. KNOX
Ever since the first documented use of spectacles for vision correction around the mid-1200s, scientists have been looking for better ways to correct human vision. With the invention of the laser, it was quickly realized that laser light can damage materials. In fact, the fields of laser micromachining and nonlinear optics emerged from these discoveries in the early 1960s.
Once considered a nuisance or source of system limitations, short-pulse laser-induced damage has now evolved into a very important area of research, development, and numerous applications. Of particular interest to the ophthalmology community is the case of femtosecond laser micromachining applied to transparent materials. Early work in systematically studying effects of laser pulse width and other properties in silica glasses1 marked the beginning of the process of turning femtosecond laser micromachining into a useful tool.
Although many excellent reviews have been written about the physics of femtosecond micromachining in various materials,2 the focus here is on the application of this process to ophthalmic materials—and more specifically for the case of localized refractive-index modification. The localization in three dimensions comes about as a result of the nonlinear nature of the multiphoton absorption process that occurs when using femtosecond lasers in the transparency range of the materials. With careful calibration and control of the excitation and writing processes, new approaches to human vision correction are made possible.
It was shown in 1999 that femtosecond lasers could be used to micromachine corneal flaps by focusing laser pulses in the range of 2–4 µJ into the stromal region of the cornea. The resulting structural modifications to the cornea could either be used to open a flap followed by tissue vaporization with an excimer laser—a process called laser-assisted in situ keratomileusis (LASIK)—or to create a lenticular section that could be extracted in a procedure called small incision lenticule extraction (SMILE).3 The greater precision and control afforded by femtosecond flap cutting was quickly adopted by the ophthalmic community, replacing the former mechanical blade, or microkeratome.
It is remarkable that despite the great success of LASIK, to date only about 2% of those needing refractive correction of some sort have actually had the LASIK procedure. Clearly, there is a market for less-invasive kinds of procedures not involving cutting of tissue.
While femtosecond micromachining is typically directed toward cutting, drilling, vaporizing, or otherwise making significant structural modifications to materials, some work demonstrated the ability to operate below a certain material damage threshold, but induce localized refractive-index modifications instead. For instance, waveguides can be written directly inside a glass block through small but well-controlled refractive index changes,4 and later it was found that refractive modification could be achieved with just a laser oscillator producing only nanojoule pulse energies.5
With this background and motivation, I started investigating how femtosecond laser oscillators could induce purely refractive index modifications below material damage threshold in a variety of ophthalmic materials in 2003. Starting initially in ophthalmic hydrogels commonly used to make intraocular lenses and contact lenses, our team found that indeed, we could write refractive-index corrections of significant magnitude (up to ±0.06) under various laser-writing conditions,6 and now see at least three major application areas for human vision correction.
Contact lenses
The industry is always looking for new ways to manufacture contact lenses at lower cost and with more versatility and novel features. For contact lens manufacturers to provide prescriptions in adequately accurate increments, they must keep more than 20,000 different kinds of lenses in stock (various combinations of sphere power, astigmatic power, and cylinder axis), each requiring custom tooling and long design cycles. We have found that by writing phase-wrapped Fresnel-type refractive correctors into hydrogels such as those used in commercial contact lenses, we could create high-quality vision correctors that, when tested in humans, show great potential for custom vision correction.7
For one such structure written into a commercial Johnson & Johnson (New Brunswick, NJ) Acuvue2 contact lens, the refractive-index change that is induced is large enough to create fully 2π phase-wrapped Fresnel structures. However, the induced reflectivity from the structure is very small, difficult to see, and photograph (see Fig. 1). The Fresnel lens had a measured power of +2.0 diopters (+2.0D) when written into a plano (no power) contact lens, and we have shown that we can write such structures in the range of -9.5 to +5D in hydrogel materials.7
There is great interest in designing new kinds of multi-focal, special-purpose, and customized contact lenses, as well as the potential to revolutionize contact lens manufacturing itself. We have found that the induced refractive index effects are effectively permanent, lasting at least five years while maintaining high image quality (see Fig. 2).Intraocular lenses
As we age, our natural human crystalline lens can undergo chemical and physical changes—in many cases resulting in cloudy vision. This medical condition is commonly referred to as cataracts.A very well-developed medical procedure is able to provide quick relief for cataract sufferers by surgically removing the old cloudy lens and replacing it with a new polymer lens. Although these procedures general go well, the desired optical correction can be in error by as much as ±0.5D, which is clinically significant for vision correction. This could be because of incorrect placement of the lens in the capsule, shrinkage of the capsule, or simply through procedural error.
In our recent materials study to optimize the amount of optical phase shift obtainable in hydrogel materials, we found that the locally induced chemical changes can be rather significant, with large observed phase shifts as a result of redistribution of water.8 Fresnel lens structures have been written into a hydrophilic (high-water-containing) lens (see Fig. 3). Another group has written phase-wrapped structures into hydrophobic (low-water-containing) intraocular lens materials9 that have been implanted into rabbit eyes—a necessary step in proving feasibility for human operations.
Direct-write cornea
In 2006, we started looking into whether the kinds of refractive-index modifications possible in ophthalmic hydrogels could be achieved in a direct-write cornea application. Experiments proved that refractive-index changes up to +0.037 could be induced in cornea from different species, including porcine, leporine, feline, and human cadaver tissue using 405 nm femtosecond laser pulses at 80 MHz repetition rate.10
Using a flat applanation procedure similar to LASIK flap cutting, we have shown that Fresnel-type 2π phase-wrapped lenses can be written directly into live cat eyes (see Fig. 4). Immediately after the writing process and removal of the applanator, a thin bubble layer is visible, indicating where the Fresnel lens was written (see Fig. 4). This layer dissipates in 10–20 minutes, leaving a clear refractive-index structure that is barely visible in specular reflection. We are able to measure the induced refractive correction by measuring the retro-reflected wavefronts, and have found that the induced optical power correction is stable for more than 18 months in live cats.
Our research indicates that, after the femtosecond laser beam has excited the tissue, the collagen fibrils in the corneal stroma are intermixed with the extracellular matrix to form a new configuration in a densified region with higher refractive index. Apparently, it is difficult for the living biology to figure out how to change the corneal structure back to its original form; therefore, it is stable.Because refractive effects induced in human cadaver tissue are comparable to those in cats, we can now contemplate direct writing in live humans. This laser-induced refractive-index change (LIRIC) process is being implemented by the startup company Clerio Vision (Rochester, NY) and scaled-up equipment is being developed for live human testing, with results to be reported in future clinical trials.
As I predicted in a Laser Focus World article in 1996, “Practical lasers will spawn varied ultrafast applications.” The nonlinear optical effects of femtosecond laser micromachining for human vision correction is just one such application that is advancing the ophthalmic industry.
ACKNOWLEDGEMENTS
Wayne H. Knox is also chief science officer at Clerio Vision, where he holds founder’s equity, but has no fiduciary or management responsibility. This research has been supported by Bausch and Lomb, the National Science Foundation Phase 1 and 2 STTR programs, the New York State CEIS Program, and a research grant from Clerio Vision. The author thanks the many collaborators and graduate students who have contributed to this work over the years.
REFERENCES
1. D. Du et al., Appl. Phys. Lett., 64, 23, 3071–3073 (1994).
2. R. R. Gattass and E. Mazur, Nat. Photonics, 2, 219–225 (2008).
3. T. Juhasz et al., IEEE J. Select Topics Quantum Electron., 5, 4, 902–910 (1999).
4. K. M. Davis et al., Opt. Lett., 21, 21, 1729–1731 (1996).
5. C. B. Schaffer et al., Opt. Lett., 26, 2, 93–95 (2001).
6. L. Ding et al., Opt. Express, 14, 24, 11901–11909 (2006).
7. G. A. Gandara-Montano et al., J. Vis., 17, 7, 38 (Jun. 2017).
8. G. A. Gandara-Montano et al., Opt. Mater. Express, 7, 9, 3162–3180 (2017).
9. R. Sahler et al., J. Cataract Refract. Surg., 42, 8, 1207–1215 (2016).
10. S. MacRae et al., “Ablation-free corneal refractive correction via ultrafast femtosecond laser induced refractive index modification,” American Academy of Ophthalmology Annual Meeting, New Orleans, LA (Nov. 2017).
Wayne H. Knox is professor of Optics and Physics, Visual Science, and Materials Science at the University of Rochester, Rochester, NY; e-mail: [email protected]ester.edu; www.whknox.com.