Photonics Products: Scientific CMOS Cameras: sCMOS cameras reach new levels of capability
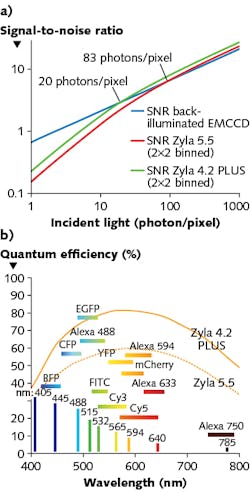
When CMOS cameras first came on the market, their imaging capabilities were too basic for many precision uses in science and industry. Over the years, however, CMOS imaging technology has grown in efficiency, dynamic range, frame rate, and signal-to-noise ratio, resulting in the scientific CMOS (sCMOS) sensor—and sCMOS technology is still advancing.
While back-illuminated electron-multiplying CCD (EMCCD) cameras are optimum for certain uses that require the lowest noise and dark currents, sCMOS technology, with its higher pixel count and lower cost, can be the best choice for a wide range of high-precision applications. sCMOS devices can capture data in a global-shutter “snapshot” mode over all the pixels or rectangular subsets of pixels, and can also operate in a rolling-shutter mode.
Andor Technology (Belfast, Northern Ireland), which makes both sCMOS and EMCCD cameras, as well as front-illuminated interline CCDs, has done extensive comparisons of these technologies. The company’s conclusion is what you’d expect—that each type of device has its own advantages (see Fig. 1).1
“Scientific CMOS (sCMOS) technology stands to gain widespread recognition across a broad gamut of demanding imaging applications, due to its distinctive ability to simultaneously deliver extremely low noise, fast frame rates, wide dynamic range, high quantum efficiency, high resolution, and a large field of view,” Andor’s comparison states. Data taken by Andor show that sCMOS cameras outperform interline CCD cameras in most key applications. Only the EMCCD camera has the edge over sCMOS, and that mainly for “extremely low light applications that require absolute raw sensitivity at respectably fast frame rates.” Andor makes two front-illuminated sCMOS cameras: the air- or water-cooled Zyla, and the Neo, which operates at a vacuum-cooled -40°C. The Zyla camera, which comes in both 4.2 and 5.5 Mpixel sizes, is intended for life-science and physical-science applications. The quantum efficiency (QE) is optimized for all fluorophores, and reaches 82% for the Zyla 4.4 and 60% for the Zyla 5.5. The devices have a 0.9 e- read noise, a water-cooled dark current of 0.019 e-/pixel/s, a 33,000:1 dynamic range, and a 100 frames/s capability, which drops to 53 frames/s when sending data via USB 3.0. The 5.5 Mpixel Neo camera has a dark current of 0.007 e-/pixel/s because of its vacuum cooling.
Back-illuminated sCMOS
Princeton Instruments (Acton, MA) produces a back-illuminated sCMOS camera, called the Kuro, which is available in 1200 × 1200 and 2048 × 2048 pixel resolutions. “This next-generation sCMOS camera significantly reduces the fixed-pattern noise seen in front-illuminated sCMOS cameras,” says Mike Melle, imaging product manager at Princeton Instruments. He notes that the Kuro has a 100% fill factor without using microlenses, which “allows for detection of photons from the UV to the NIR without a reduction in quantum efficiency,” according to Melle.
The Kuro 2048B has a QE of >95% at 550–600 nm wavelengths (see Fig. 2) and a readout noise of 1.3 e- root mean squared (RMS). The camera delivers 42 frames/s at full resolution and 100 frames/s at a 1200 × 512 pixel resolution, useful for high-speed applications such as live cell imaging. In addition to applications in microscopy and fluorescence, the Kuro camera is aimed at a number of uses in astronomy, including adaptive optics, lucky imaging, speckle imaging, exoplanet searches, tracking near-earth objects, and solar astronomy.High dynamic range
A sCMOS camera produced by Thorlabs (Newton, NJ), the Quantalux, is intended for imaging applications such as fluorescence microscopy, night vision imaging, marine biology, bioluminescence, materials inspection, multispectral imaging, or fluorescence in situ hybridization (FISH). It has a read noise of 1e-, a 2.1 Mpixel 1920 × 1080 resolution, and full-sensor frame rates up to 50 frames/s. The unit has a QE of 61% and a read noise of 1 e-. The sensor and electronics are packaged in a 2.38 × 1.88 × 2.78 in. housing that provides passive thermal management for the sensor so as to minimize the increase in the dark current without using a cooling fan or thermoelectric cooler.
“Under low-light imaging conditions, the camera must generate a signal that is large enough to overcome noise in order to obtain a discernible image,” says Ash Prabala, chief technologist and co-manager at Thorlabs Scientific Imaging. “When relatively few signal photons are collected during a relatively short exposure, it is the read noise that is the dominant source of noise. Under these conditions, one seeks a sensitive camera with low noise: a camera with higher QE and lower read noise is most desirable. A camera with higher QE-to-read-noise ratio will produce a higher signal-to-noise ratio (SNR) under low-light conditions (all else being equal).”
A common problem in scientific imaging involves imaging bright features in the presence of a dim background, Prabala notes. “If the camera doesn’t have a large enough dynamic range, saturation and floor limitations will limit the simultaneous capture of both bright and dim details,” he explains. “With the Quantalux camera’s high dynamic range of 87 dB, a single exposure gathers sufficient contrast of bright, moderate, and dim objects at once. We worked with Craig Brideau of the University of Calgary in Alberta, Canada to demonstrate this with images of an approximately 20-µm-thick slice of 5xFAD mouse with amyloid plaques stained with Thioflavin S (see Fig. 3).”Space-debris tracking
Ximea (Münster, Germany) is another company that makes both CCDs and sCMOS cameras—the sCMOS sensors are thermoelectrically cooled to -20°C.
“In regard to sCMOS, Gpixel is our primary vendor for the sensor that is ideal for low light, high-energy physics, x-ray tomography, energy-dispersive direct x-ray detection, astronomy, microscopy, and so on,” says Kevin Toerne, sales engineer at Ximea. Applications that are photon-starved and/or require long integration times are the ones that will benefit, he notes.
Ximea’s xiJ camera line, which includes a 4.2 Mpixel model (GSENSE2020BSI Backside illuminated sensor), has a read noise of 2e-. Backside illumination provides QE levels of 95% that allow for detection of very faint emissions, and the low temperature of the sensor helps with long exposures, Toerne explains.
“All this coupled with high speed of CMOS technology for a quick readout of 40 frames/s and faster. The camera also offers large dynamic range by using dual amplifiers for each pixel,” he says, adding that the dynamic range, high sensitivity, and fast readout makes the camera well suited for applications such as space-debris tracking to better predict orbital periods.
sCMOS excels for microscopy
The associated companies Photometrics (Tucson, AZ) and QImaging (Surrey, BC, Canada) offer CCD, EMCCD, and
sCMOS cameras for life-science research, and have done comparison of the various camera types.2 The two companies’ sCMOS cameras fall into two product lines, the Prime and the Iris series, as described by Daniel Croucher, applications team manager.
The Prime sCMOS is a front-illuminated camera with 72% QE, 6.5 µm pixels, 100 frames/s speed, 4.2 Mpixels (2048 × 2048), and an 18.8 mm diagonal field of view. The Prime BSI is a back-illuminated version of the Prime sCMOS, boosting the QE to 95%. It has a 63 frames/s speed. The Prime 95B is also back-illuminated (95% QE), but differs from the Prime sCMOS and BSI in other specifications: 11 µm pixels, 82 frames/s speed, 1.44 Mpixels (1200 × 1200), and an 18.66 mm diagonal field of view.
“The Prime sCMOS can be used for a broad range of fluorescence microscopy applications from high light to somewhat low light such as widefield imaging, light sheet microscopy, cellular imaging, microfluidics and calcium imaging,” Croucher says, “but it will struggle at very low light. The Prime BSI is the natural next step up from the Prime sCMOS, as the quantum efficiency has been improved from 72% to 95% without changing the other specifications. This means the camera has higher sensitivity, so gives higher performance for low-light imaging applications.”
The higher sensitivity of the Prime BSI allows it to be used in superresolution localization microscopy (PALM, STORM, DNA-PAINT), single-molecule imaging, spinning disk confocal, and TIRF microscopy, Croucher notes. “The Prime 95B is also back-illuminated, but has a larger pixel size (11 µm) than the BSI. This makes it even more sensitive and an EMCCD replacement,” he says. “This camera is designed for the lowest-light applications and is the preferred choice for spinning disk confocal microscopy, TIRF microscopy, superresolution localization microscopy (PALM, STORM, DNA-PAINT), SIM superresolution microscopy, and single molecule imaging.”
The Iris series of CMOS cameras have 73% QE, 4.25 µm pixels, and a 30 frames/s speed. The Iris 9 has 9 Mpixels (2968 × 2968) and a 17.8 mm diagonal field of view, whereas the Iris 15 has 15 Mpixels (5056 × 2968) and a 25 mm diagonal field of view.
“The real advantage of the cameras comes from the smaller pixel size (4.25 µm) for higher-resolution imaging, which better suits lower-magnification objectives,” Croucher says. “The other major advantage is the massive field of view offered by the Iris 15.” The applications for both Iris cameras are largely the same—widefield fluorescence, multicolor fluorescence, light-sheet microscopy, cellular imaging, microfluidics, and high-content screening.
The laboratory of Eli Rothenberg at the New York University School of Medicine (New York, New York) focuses on new optical methods to study biological molecules and processes at real-time and nanometer-scale (see Fig. 4).3 One of the challenges of imaging replicating DNA is that inside the nucleus of the cell, there are many labeled components crowded together, as well as very small components that need to be clearly resolved.The researchers moved from EMCCD technology to the Prime 95B back-illuminated sCMOS camera. “For single-molecule localization imaging, the most important thing is the reconstruction process in how we fit each single-molecule point spread function into its centroid coordinate,” says Yandong Yin, one of the researchers, noting that more than 90% of the pixels in Photometrics’ camera fall within a very tight noise distribution.
Another type of low-light CMOS
The sensor in the low-light CMOS camera made by Photonis (Mérignac, France; U.S. office in Sturbridge, MA) is different from the sensors used by many of the other companies in the sCMOS industry, as many companies use the same sensor source, according to Margaret Cooley, international marketing manager at Photonis. “Our sensor is proprietary to us,” she notes.
“There are significant technical differences, most noticeably in the pixel size: 6.5 µm for the [standard] sCMOS and 9.7 µm for the Photonis sensor,” Cooley says. “Our sensor is most useful in low light conditions where a broad-bandwidth response is required from the sensor—we cover 350–1100 nm.” This makes the Photonis sensor more useful for day-through-night surveillance with IR illumination detection than for science applications, she explains.
“However, we are currently looking at endoscopic techniques where a much-smaller fiber guide (therefore, a smaller incision) can be used; the extreme sensitivity in low light can still provide significant detail and without the need for cooling,” Cooley says, although she does note that the size of the sensor (1280 × 1024 pixels) and the standard video frame rates (50/60 Hz) do not make the sensor ideal for superresolution fluorescence applications where the frame count is in the thousands per second.
Photonis’ color Nocturne camera, which contains the proprietary KAMELEON sensor, has frame rates to 100 Hz and read noise of 4 e-.
REFERENCES
1. See https://goo.gl/p2qKw8.
2. See https://goo.gl/UD7nCT.
3. See https://goo.gl/KHa5Cb.
For More Information | |
Companies mentioned in this article include: | |
Andor Technology | Princeton Instruments |
Photometrics | Thorlabs |
Photonis | Ximea |
DISCLAIMER: While we try to include information from the broadest possible number of companies that manufacture the products featured in our Photonics Products series, because of limited word count as well as deadlines that cannot always be met by requested contributors, we cannot possibly include all companies and regret if your company is not included in our series. |
About the Author
John Wallace
Senior Technical Editor (1998-2022)
John Wallace was with Laser Focus World for nearly 25 years, retiring in late June 2022. He obtained a bachelor's degree in mechanical engineering and physics at Rutgers University and a master's in optical engineering at the University of Rochester. Before becoming an editor, John worked as an engineer at RCA, Exxon, Eastman Kodak, and GCA Corporation.