Photonics Products: Lidar Systems: Automotive lidar draws heavily on photonics industry
A self-driving car or other vehicle is a complex thing, combining light-based and/or other sensors, GPS, other dedicated hardware, and sophisticated software that together allow the vehicle to safely navigate its surroundings and nearby pedestrians to remain in one piece. The same forms of sensing can also be used for automatic braking and vehicle avoidance in vehicles that are not entirely autonomous.
Automotive lidar is just one form of sensor (radar and intelligent vision are two others) that can map an autonomous vehicle’s surroundings. However, because of the numerous lidar startup companies, as well as billions of dollars of R&D and investment capital, automotive lidar is a very visible marker of how fast autonomous-vehicle technology is advancing.
Many technical approaches to automotive lidar exist, including various types of scanning, phased arrays, and pulsed camera-based flash. However, the purpose of this article is not to do a survey or even a thorough description of these approaches, as such information could fill a book. Instead, what we will do here is to, by example, show the large overlap between the automotive lidar industry and the photonics industry so heavily covered by Laser Focus World (this article assumes a working knowledge of lidar concepts). Many, if not most, of the commercial outfits and their products appearing in this article will be familiar to our audience—and that is a good thing, as it shows how central our industry is to the future of applied high technology.
Producing and detecting the signal
Photonics companies involved with automotive lidar may produce either complete lidar systems, one specific type of lidar component, or a range of components. In an example of the last, Hamamatsu (Hamamatsu City, Japan) provides a wide variety of photonic components for a lidar system from design to assembly/testing to manufacturing, light sources and photodetectors for emission and detection of light, as well as beam-control components such as microelectromechanical systems (MEMs) mirrors and fast-axis collimating (FAC) lenses for laser diodes, according to Jake Li, business development manager of auto lidar at Hamamatsu.
The company’s automotive-grade photodetectors are silicon- and indium-gallium-arsenide (InGaAs)-based to cover the need for lidar designs from 800 to 1600 nm, with enhanced sensitivity at 905 and 1550 nm that improves the detection range of lidar designs, Li notes. For light sources, Hamamatsu focuses on providing 800- to 905-nm-based laser diodes that are single- to three-stack designs for the high-power output needs of any lidar design, and are offered in continuous-wave (CW) or pulsed (PLD) mode. “To enable a 3D lidar system design, we provide various beam-control components,” Li says. “Hamamatsu’s 1D or 2D automotive-grade electromagnetic based MEMs mirrors operate in a combination of linear and/or resonant modes, which can steer the beam of light to various directions. Our FAC collimates light spreading from a semiconductor laser, which is a critical component to control the amount of light divergence in a lidar system.”
For a lidar system, only a small fraction of the emitted photons from the target are detected. Therefore, selecting the right photodetectors with high detection sensitivity, high internal gain, and lower noise and cap is critical. For 905 nm short- to long-range lidar concepts, silicon avalanche photodiodes (APDs) and silicon photomultipliers (SiPMs) are popular detectors—each has specific advantages and limitations, Li says. For long-range detection, eye-safety concerns resulting in range limitations of some lidar concepts has illustrated the importance of 1550 nm lidar systems. For these systems, Hamamatsu provides InGaAs-based photodetectors such as InGaAs photodiodes or APDs. “Hamamatsu’s continued innovation should mitigate some critical disadvantages of this technology, which are higher cost and lack of array offering,” Li says.
Fast acquisition and detection are needed to generate a high-density point cloud. This can be accomplished by using an array of photodetectors, where each photodetector samples a distance to a particular scene point. “Traditionally, APD arrays were used,” Li says. “However, an APD requires a high biasing voltage (more than 150 V) and a temperature-compensation circuit. These two requirements increase power consumption, which is undesirable in a self-driving vehicle with a limited amount of available power. SiPM resolves this problem, since both temperature sensitivity and bias voltage are significantly lower.”
Two lasers optimized for different lidars
Lumentum (Milpitas, CA) produces a range of diode lasers (the term “diode laser” is sometimes used instead of “laser diode” to refer to a complete laser as opposed to a component such as a diode chip), including lasers for long- and short-range lidar.
As described by Tomoko Ohtsuki, product line manager at Lumentum, the company offers two key components for lidar: 1550 nm narrow-linewidth distributed Bragg reflector (DBR) diode lasers optimized for coherent lidar, and high-power 940 nm vertical-cavity surface-emitting laser (VCSEL) arrays. Ohtsuki says that Lumentum diode lasers have a track record of 200 million delivered units and a failure rate of less than one part per million.
The 940 nm VCSELs are optimized for high-resolution flash lidar and in-cabin monitoring, and the 1550 nm narrow-linewidth DBR diode lasers are suitable for coherent frequency modulated continuous-wave (FMCW) lidar designs. “Particularly important for long-range lidar, DBR diode lasers are optimized for lidar at greater than a 200 m range, and 1550 nm wavelength offers 100 times the power of shorter-wavelength lasers with improved eye safety compliance,” Ohtsuki notes.
Narrow-linewidth DBR diode lasers in coherent FMCW systems can be used for next-generation long-distance lidar systems that require less power, providing the ability to simultaneously detect velocity and distance, Ohtsuki adds. Lumentum’s narrow-linewidth DBR diode lasers use compact indium phosphide (InP) laser diodes long used for telecom optical communications and have inherent noise filtering and rejection of out-of-band wavelengths.
Lidar with silicon photonics
Blackmore (Bozeman, MT) makes complete coherent frequency modulated continuous-wave (FMCW) lidar systems with ranges greater than 200 m (see Fig. 1). The FMCW concept combines modern coherent optical telecommunications hardware with advanced radar signal-processing techniques.
“Blackmore extracts and assembles range and Doppler data into point clouds in real time. This allows points to be streamed from the sensor with submillisecond latency,” explains Stephen Crouch, the company’s cofounder and CTO. “Its systems use coherent detection to make measurements with single-photon sensitivity limited only by quantum noise, which helps see through rain, snow, and dust. They also deliver extremely high dynamic range and can see both bright objects, such as street signs and tail lights, as well as darker ones, like tires and road surfaces, with ease.”
The systems’ real-time computations allow for processing on commodity embedded processing hardware. As a result, the company is developing application-specific solid-state scanners in silicon photonics to pair with Blackmore’s lidar engine for the mass manufacture of completely solid-state lidar sensors, Crouch says.
Direct-detection lidar systems cannot simultaneously measure range and Doppler (velocity information), Crouch notes. Compared to amplitude-modulated pulsed lidar, Blackmore’s Doppler lidar senses real-time velocity of objects at long distances, giving autonomous vehicles a number of critical benefits, including immunity to interference from sunlight as well as from other sensors (including other lidar sensors), and rapid, simple, and direct measurement of velocity, in contrast to the computationally intense and slower approach used by traditional pulsed lidar systems, in which the velocities of objects are calculated using multiple frames of data.
Crouch says that Blackmore’s interference-free Doppler lidar sensors produce a unified point cloud (selectable point throughput of 300 kpts/s to 1.2 Mpts/s) with instantaneous velocity on every measured point (±150 m/s with a 0.2 m/s resolution).
Doppler velocity measurements can help to provide insight into pedestrian intent, especially in dense urban environments (see video at https://goo.gl/KdBzw8). The Doppler data field can also act as an independent input into vehicle localization via analysis of the relative velocity between static objects and a moving car with the lidar. Doppler data is also useful to filter noisy points in rain, snow, and dust conditions.
Developers’ tools included
Another company providing a complete long-range (up to 250 m), high-resolution automotive lidar platform is Luminar (Orlando, FL). According to Jason Eichenholz, the company’s cofounder and chief technology officer, the platform’s primary subsystems—the laser, the scanner, and the receiver—have all been developed by Luminar from the chip level up to achieve the performance necessary to enable safe and ubiquitous autonomy in highway and urban driving applications. For example, the receiver technology was developed from the InGaAs chip through the silicon ROIC and electronic architecture.
In the lidar platform, long-range and high-resolution 3D sensing is combined with high-fidelity target-geometry detection to classify objects over range. Point attributes (for example, reflectance) are also captured to quickly segment and classify objects over range (see Fig. 2).“The sensor must be sensitive enough to reliably measure small signal returns from distant, uncooperative targets while enduring high energy returns from nearby retroreflectors or other high reflectivity targets,” Eichenholz says. “At the core of Luminar lidar is a highly sensitive hybrid receiver mating discrete and low-cost InGaAs die with mature and sophisticated silicon logic. The lidar system leveraging this receiver is capable of ranging millions of points per second, each capable of more than 250 meters of ranging capability.”The sensing platform has a pixel density of 250 points per square degree of resolution towards the horizon of the image (current systems deployed now will do about 5 points per square degree), according to Eichenholz.
Luminar has delivered developer tools to partners that aid their self-driving stack software development with 3D lidar data infrastructure, labeling, and annotation tools, Eichenholz notes. “These tools allow partners to take full advantage of the high-fidelity data,” he says. “This perception platform is able to demonstrate new layers of detail in the field of view and is capable of charting the velocity, movement, and detection of objects in view.”
Lasers in many forms
It would make perfect sense if a large optoelectronics maker with expertise in light sources were interested in automotive lidar. Indeed, Osram (Munich, Germany), best known for its lighting and other LEDs, is in the game. As described by Rajeev Thakur, regional marketing manager at Osram, the company provides lasers for lidar that have a 905 nm wavelength and peak power per channel up to 120 W at 40 A.
“We offer these lasers in many forms, from bare die to plastic package and in future in surface mounted quad-flat no-leads (QFN) packages,” Thakur says. “Current lasers offered are single channel; in [the] future, we will offer multichannel lasers. In addition, we offer photodiode detectors, which work well with our 905 nm lasers.”
Currently, Osram’s single-channel bare die (SPL DS90A_3) is widely used by major lidar manufacturers, Thakur notes. The bare die has a small form factor (0.4 × 0.6 mm), with three vertically stacked lasers producing a peak power of 120 W at 40 A. The spectral width is ±5 nm and the operating temperature is from -40° to 105°C—the maximum permitted duty cycle is 0.1%.
“The qualification for the SPL DS90A_3 will be performed referenced to typical automotive operating conditions,” Thakur says. “We have over 10 million lasers in the field with over 10 years of field exposure with zero chip failures to date.” The key reason that customers like the emitter, apart from robustness, Thakur adds, is the high peak power (120 W), small aperture (200 µm), and small form factor (it allows densely integrated multiple lasers to be stacked up as needed for the design). He says that many scanning rotating lidars use these emitters, as the high peak power provides a signal that is capable of achieving a lidar range of 200 m with low-reflectivity (10%) objects.
“Phantom Intelligence (Québec City, QC, Canada) has developed an automotive lidar platform using Osram’s infrared (IR) pulsed laser diodes and photodiodes that work to detect objects on the road and help prevent a collision,” Thakur says. “The lasers deliver a high efficiency of up to 40% at 70 W peak output power, achieving a long operating lifetime.”
Micropolarizer camera
Resources in the photonics industry with regards to intellectual property (IP) are vast. For autonomous vehicles, this means that technology developed for some other purpose may turn out to be ideal for lidar. As an example, Neal Brock, director of new technology development at 4D Technology (Tucson, AZ), notes that researchers have shown that micropolarizer cameras can improve performance and reduce complexity of flash lidar systems.
The micropolarizer camera technology was originally developed by 4D Technology several years ago for use in dynamic interferometers says Brock. A micropolarizer camera is similar to a color camera, except that it senses the polarization of light instead of the color of light. Instead of the red, green, and blue pixel pattern of the color camera, a pixel pattern of four different polarizers is used in the micropolarizer camera (see Fig. 3). As with the color filters of a color camera, the polarization filters of the micropolarizer camera add no additional weight or bulk to the camera, which is virtually indistinguishable from a monochrome camera. Micropolarizer cameras with array sizes as large as 5 Mpixels are available. Several lidar research groups are developing lidar systems using micropolarizer cameras. Recently, a research group with the Korea Advanced Institute of Science and Technology (KAIST; Daejeon, South Korea) demonstrated the advantages that micropolarizer camera technology can offer to lidar systems.Most lidar systems measure the time-of-flight (ToF) of a rapidly pulsed laser beam that is rapidly scanned across the imaged scene. The systems are often bulky and have relatively low spatial resolution. The relatively simple lidar system that was demonstrated, which has no moving parts, uses a 1 Mpixel 4D Technology micropolarizer camera, a pulsed laser, and a Pockels-cell-based polarization modulator to rotate the polarization of the light as it returns from the scene. The laser illuminates the entire scene in a single short pulse of light. The Pockels cell, which is synchronized with the laser pulse, rotates the polarization of the light as it returns from the scene such that its arrival time corresponds to a unique polarization or retardation. The micropolarizer camera captures, in a single video frame, the pulse of light from the scene that has been temporally coded with polarization. The four polarizations instantaneously captured by the micropolarizer camera enable the phase retardation (and distance) to be calculated at every point in the scene.
Laser-diode collimating optics
LightPath Technologies (Orlando, FL), a company that produces IR and visible optics via different fabrication processes including molding and diamond turning, has numerous types of optical capabilities relevant to automotive lidar, according to material provided by Andrew Locke, applications engineering manager. Locke notes in particular the company’s expertise in collimator lenses and assemblies of various types.
Precision glass molding enables the low-cost large-scale production of laser-diode collimation lenses that are anamorphic, allowing them to precisely collimate the beam of a laser diode with beam divergences (and even source focus position) that differ in its two orthogonal axes. In addition, the capability of high-volume manufacture of molded lenses with other asphere and freeform surfaces is there if needed.
LightPath also makes fused-fiber collimators, in which the end of a glass optical fiber that carries high-power laser light is fused to one end of a monolithic glass lens matched in refractive index, eliminating any chance of misalignment, as well as two glass/air surfaces that could induce optical loss. Rounding out the company’s offerings are air-gap and hybrid fiber collimators that, along with an air gap, include a window fused to the fiber end to reduce the chance of fiber-end damage.
MEMS scanning
For scanned lidar applications, rapidly and reliably steering laser beams into a target environment can be achieved using dual-axis MEMS mirrors (see Fig. 4), as in the scanning systems produced by Mirrorcle Technologies (Richmond, CA). These mirrors, even with simple metallic coatings available in current products, can handle the variety of wavelengths, pulsed or CW powers, that are used in most lidar designs, according to Abhishek Kasturi, senior electronics engineer at Mirrorcle. When designed with electrostatic actuation and purely silicon construction, MEMS mirrors have been proven to have extremely wide environmental temperature tolerance, good reliability, and repeatability.“Monostatic dual-axis designs require larger apertures and larger angles,” Kasturi says. “Thus, we manufacture large-diameter, large-angle dual-axis MEMS mirrors, compact MEMS drivers, electronic controller units, and software to facilitate driving, synchronizing with receivers, and so on.” Typical scan rates are >500 lines/s in this category.
In duostatic designs, the outgoing laser beam and the receiver optics are two separate apertures, allowing the MEMS mirror size to be smaller and therefore faster, with a higher bandwidth and highest robustness in shock and vibe. These devices are suitable for customers with camera-based detection systems or classic ToF receivers, and have smaller diameter but larger-angle dual-axis MEMS mirrors. Typical scan rates are >2000 lines/s, but can reach >20000 lines/s scan rates with a design containing two single-axis MEMS mirrors. “Arrayed-emitter designs are essentially in line with Velodyne’s (San Jose, CA) spinning array of individual lidars, where the spinning motor can be replaced with a single-axis MEMS mirror for a more compact and cost-effective solution, albeit with a smaller range of azimuth angles,” Kasturi explains. Two designs address this space with rectangular “line” mirrors. The faster and smaller area mirror (4 × 1.3 mm) can be used at any scan rates up to at least 3500 scans/s. The largest mirror in this category (6 × 2.4 mm) can be used at up to 800 scans/s. Mirrorcle also produces scan modules, in which laser integration, beam forming, alignment, and driving are already taken care of.
Many scanning-based automotive lidar systems manufacturers have interacted with Mirrorcle. “While we cannot disclose most customers and their methodologies, we can present work done by Army Research Labs (ARL) in 2012 that acts as a reference design1 for various lidar systems, including Boeing’s SpectroScan 3D,”2 Kasturi says. “ARL has since updated the design with Mirrorcle’s latest MEMS mirrors along with improvements on their sensor system, and presented their progress at SPIE DCS in 2016.”3
Coated optics with multiple capabilities
Spectrally selective optical components are critical to automotive lidar systems. Viavi Solutions (Milpitas, CA) provides two main optical components of this type for lidar, as noted by Georg Ockenfuss, the company’s director of worldwide field application engineering.
“One is a patented low-angle-shift (LAS) near-infrared (near-IR) bandpass filter, which sits in front of the detector, Ockenfuss says. “Viavi optical filters address two major issues for lidar systems in self-driving car designs: by virtue of its high transmission, it allows the use of a dimmer return beam, thus enabling a light signal to travel further to its target. And because of its low angle shift, it enables the lidar system to operate at a high level of reliability in the brightest levels of sunlight. Viavi optical technology filters the return light such that the signal-to-noise ratio can be improved by as much as 150% over other more traditional filters.”
The second product, currently under development at Viavi, is an external window for lidar systems (see Fig. 5). The window has an antireflection coating (AR) for the light source’s wavelength—the coating works over an angle range from -60° to 60°. “Additionally, this AR can be black or any color in the visible spectrum for more pleasing aesthetics to the automobile designer,” Ockenfuss says. “The window is opaque in the visible spectrum, which also keeps the technical components behind it invisible to a human. The front side of the window is coated with highly durable hydrophobic and oleophobic coating, preventing water condensation and allowing for easy cleaning. The back side of the window contains a transparent conductive coating, which enables the window to be heated for defrosting and de-icing.”Both the LAS near-IR bandpass filter and the durable black near-IR AR window use the same fundamental technology, which was developed almost 10 years ago by Viavi—a process to deposit silicon that is highly transparent down to wavelengths of about 780 nm. Silicon has a refractive index of about 3.7 at 900 nm, which is significantly higher than that of any oxides typically used in thin-film interference filters for the near-IR spectrum. This greatly expands the design space for near-IR thin-film filters.
REFERENCES
1. R. Moss et al., “Low-cost compact MEMS scanning ladar system for robotic applications,” Proc. SPIE, 8379, 837903 (2012).
2. See https://goo.gl/7KVE5D.
3. B. L. Stann et al., “Progress on MEMS-scanned ladar,” Proc. SPIE, 9832, 98320L (2016); doi:10.1117/12.2223728.
For More Information
Companies mentioned in this article include:
4D Technology
Bedford, NH
Blackmore
Bozeman, MT
Hamamatsu
Hamamatsu City, Japan
LightPath Technologies
Orlando, FL
Lumentum
Milpitas, CA
Luminar
Orlando, FL
Mirrorcle Technologies
Richmond, CA
Osram
Munich, Germany
Phantom Intelligence
Québec City, QC, Canada
https://phantomintelligence.com
Velodyne
San Jose, CA
Viavi Solutions
Milpitas, CA
DISCLAIMER: While we try to include information from the broadest possible number of companies that manufacture the products featured in our Photonics Products series, because of limited word count as well as deadlines that cannot always be met by requested contributors, we cannot possibly include all companies and regret if your company is not included in our series.
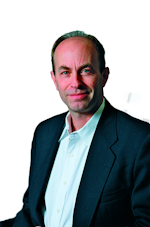
John Wallace | Senior Technical Editor (1998-2022)
John Wallace was with Laser Focus World for nearly 25 years, retiring in late June 2022. He obtained a bachelor's degree in mechanical engineering and physics at Rutgers University and a master's in optical engineering at the University of Rochester. Before becoming an editor, John worked as an engineer at RCA, Exxon, Eastman Kodak, and GCA Corporation.