It is impossible to understate the importance of noninvasive soft tissue imaging in modern medicine, with ultrasound and magnetic resonance imaging (MRI) currently being the two most popular techniques. However, while these techniques provide excellent depth penetration, typically 1 to 10 cm, the long wavelengths of acoustic and radio waves limit the image resolution to a roughly 1 mm voxel. By contrast, optical imaging techniques such as optical coherence tomography (OCT) offer micron-scale voxels along with molecular specificity. But the high scattering cross-section of biological tissue limits most optical detection methods to a maximum of 1 mm depth penetration.
In the mid-1990s, radiologists at the Indiana University Medical Center (Indianapolis, IN) proposed a new method called photoacoustic ultrasound reconstruction tomography to provide optical imaging with ultrasonic penetration depth.1 While promising, the technique now known more broadly as photoacoustic imaging (PAI) remained fairly under the radar for the following decade. When Paul Beard from University College London (London, UK) published a critical review of PAI in 2011,2 he pointed out that, “This early work, undertaken by a handful of researchers, progressed steadily, if not with any notable degree of rapidity.” He further explained it was not “until the early- to mid-2000s when the first truly compelling in vivo images began to be obtained.”
While some early clinical work had been performed by that time, PAI was still primarily used as a tool for basic biological research. Over the subsequent decade, PAI continued to evolve and has now been successfully demonstrated in a wide range of clinical applications—including breast, dermatological, and vascular imaging.3 2021 marked perhaps the most significant milestone when the Imagio breast imaging system (see Fig. 1) from Seno Medical (San Antonio, TX) became the first PAI system to cross the “valley of death” as the United States Food and Drug Administration (FDA) granted premarket approval.
Photoacoustic imaging foundation
To understand the potential impacts of PAI in healthcare, it is essential first to take a step back and understand the fundamentals of the technique. PAI relies on optically induced acoustic waves in soft tissue, which can then be recorded using one or more ultrasound transducers. Typical PAI systems utilize a nanosecond pulse width Q-switched laser for exciting various chromophores in the tissue, creating localized heating. Assuming the laser fluence is below the ionization threshold of the chromophore and the thermal damage threshold of the surrounding tissue, the resultant heat will be quickly dissipated through the tissue, resulting in a propagating pressure differential. This pressure gradient generates acoustic waves in the tissue upon repeated laser pulses, which are easily detected with an ultrasonic transducer.
In addition to the increased resolution compared to conventional ultrasound imaging, PAI has the advantage that chromophore absorption is wavelength-dependent. This means that using multiple excitation wavelengths, PAI can provide a degree of chemical discrimination. When combined with a broadly tunable laser source, it is possible to produce full hyperspectral subdermal images. For example, the Acuity family of multispectral optical tomography (MSOT) PAI systems from iThera Medical (Munich, Germany) utilizes a tunable laser from 660 to 1300 nm, allowing for the differentiation of most endogenous biological chromophores. While complete spectral information is helpful in clinical research applications, the long scan time currently makes this approach prohibitive for mass deployment. However, since most PAI applications are targeted at specific chromophores, full spectra imaging is generally unnecessary.
Current clinical applications
The most common endogenous chromophores used in PAI application are oxyhemoglobin (HbO2) and deoxyhemoglobin (HHb). These are particularly interesting for tumor screening due to the increased metabolic activity within cancer cells. The Imagio breast imaging system was explicitly designed for that exact purpose, combining dual-wavelength PAI with traditional ultrasound to produce a false-color overlay of blood content over a grayscale ultrasound image. This system utilizes dual 757 nm and 1064 nm excitation lasers to take advantage of the relatively high absorption of HHb compared to HbO2 at 757 nm, as opposed to 1064 nm where the relative absorption flips. From Figure 2, we can see the traditional grayscale ultrasound image of the mass in the first image, along with a map of the total blood in yellow (image 4) and the differentiated HHb in red and HbO2 in green (images 2 and 5). For the first time, this system provides clinicians with an FDA-approved real-time noninvasive means of identifying cancers without the need for ionizing radiation.PAI offers a significant improvement over MRI for vascular imaging since it does not require external contrast agents. Laser speckle contrast imaging (LSCI) can also detect subdermal blood flow,5 but it does not provide the spatial resolution needed to map the vascular architecture, nor can it monitor oxygenation. That said, it should be noted that the speed and non-contact nature of LSCI compared to PAI make it attractive for specific applications such as real-time interoperative blood flow monitoring.
The next generation of PAI systems
While most PAI systems still rely on Q-switched laser excitation, there is a growing trend towards the use of laser diodes and even LEDs. LEDs are the most attractive of these two options due to their extremely low cost, complexity, and power consumption. While it is true that LEDs can in no way match the performance of Q-switched lasers, they are still suitable for certain applications.
Yunhao Zhu et al. point out in their 2020 review of LED-based PAI6, “[i]n the span of 10 years, there has been significant growth in this field, especially with the improvement of pulse energy (nJ [nanojoules] to hundreds of µJ [microjoules]) and PRR [pulse repletion rate] (200 Hz to 16,000 Hz) of LEDs.” They further explain “that LED-PAI is now capable of functional imaging (oxygenation and blood flow imaging) of superficial and sub-surface tissue (more than 1 cm) at frame rates unachievable for laser-based systems (500 Hz).” The authors also made sure to point out the limitations of LED-based PAI, including the lack of tunability across chromophores, longer pulses reducing acoustic efficiency, and lower optical power reducing depth penetration. Still, LED-based PAI systems show great potential for use in future low-cost point-of-care or wearable instrumentation.
While most well known for their robotic hybrid assistive limb (HAL) technologies, Cyberdyne (Ibaraki, Japan) has also become one of the first companies to offer a commercial LED-based PAI system called the Acoustic X. While the Acoustic X is currently only approved for medical research purposes and is limited to a maximum depth penetration of 40 mm, it clearly shows the potential for future of LED-based PAI technology.
Another exciting development in PAI is the development of dual-mode PAI-OCT platforms. First demonstrated in 2009 by Lihong Wang’s group at Washington University in St Louis (St. Louis, MO),7 this technique combines the high resolution of OCT with the chemical specificity of PAI. To date, most of the research involving PAI-OCT has focused on microscopy-based systems, but there has also been some up-and-coming research into photoacoustic endoscopes (PAE).
It’s important to note that while extremely promising, there is still much work to be done before endoscopic PAE-OCT systems are ready for deployment. As Zohreh Hosseinaee et al. explained in a review of dual-mode PAI-OCT, “In general, future direction for multimodal PAE-OCT studies can be focused on improving scanning speed, miniaturizing the probe size, and enhancing detection mechanism.”8
It seems clear that the FDA approval of the Imagio breast imaging system is likely only to be the tip of the iceberg. With PAI gaining acceptance and researchers continuing to push the limits of the technology in the lab, it is more evident than ever that PAI is poised to revolutionize noninvasive imaging across the healthcare industry.
REFERENCES
1. R. A. Kruger, P. Liu, Y. Fang, and C. R. Appledorn, Med. Phys., 22, 10, 1605–1609 (1995).
2. P. Beard, Interface Focus, 1, 602–631 (2011).
3. A. B. E. Attia et al., Photoacoustics, 16, 100144 (2019).
4. Y. Matsumoto et al., Sci. Rep., 8, 786 (2018).
5. See https://bit.ly/3F8xBjK.
6. Y. Zhu et al., Sensors, 20, 9, 2484 (2020).
7. L. Li, K. Maslov, G. Ku, and L. V. Wang, Opt. Express, 17, 19, 16450–16455 (2009).
8. Z. Hosseinaee, J. A. Tummon Simmons, and P. H. Reza, Front. Phys., 8, 635 (2021).
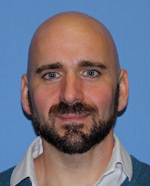
Robert V. Chimenti | Director, RVC Photonics LLC
Robert V. Chimenti is the Director of RVC Photonics LLC (Pitman, NJ), as well as a Visiting Assistant Professor in the Department of Physics and Astronomy at Rowan University (Glassboro, NJ). He has earned undergraduate degrees in physics, photonics, and business administration, as well as an M.S. in Electro-Optics from the University of Dayton. Over a nearly 20-year career in optics and photonics, he has primarily focused on the development of new laser and spectroscopy applications, with a heavy emphasis on vibrational spectroscopy. He is also very heavily involved in the Federation of Analytical Chemistry and Spectroscopy Societies (FACSS), where he has served for several years as the Workshops Chair for the annual SciX conference and will be taking over as General Chair for the 2021 SciX conference.