Imaging highlights tumor-free margins in cancer surgery
The patient lies anesthetized, two portals—small surgical openings—between two ribs. The surgeon directs thoracoscopic instruments through the portals: scissors, a grasper, a stapler, and a remote camera. Computed tomography (CT) and positron-electron tomography (PET) scans have identified a lung tumor. Now, the tough part: completely excise the tumor while removing as little healthy tissue as possible. The tumor doesn’t make it easy. Yes, some dense regions are visibly different from the healthy tissue, but marginal areas are nearly indistinguishable.
Then, the surgeon flips a switch, illuminating the tumor region (see Fig. 1). Earlier, a targeted fluorophore had been injected—it bound to tumor tissue and cleared from the unaffected tissue. The excitation light stimulates the tumor to emit longer-wavelength light. In moments, the surgeon removes the glowing tissue, knowing with nearly absolute certainty that the tumor has been successfully resectioned.
All the pieces—targeted fluorescent labels, fluorescence imaging systems, and image processing software—are available. Yet this type of surgical assistance, fluorescence-guided surgery (FGS; also known as image-guided surgery or intraoperative imaging), is rarely an option (see Fig. 2).The reasons for this, as with all new technological developments, are in the details. Yes, targeted fluorophores are available, but how much contrast do they provide, and for which tumors? Excitation sources and sensors stimulate and detect fluorescence, but can they provide actionable information? Even if those challenges are met, does FGS help patients live longer, healthier lives?
Stick and shine
The simple requirement for any FGS fluorescent agent is that it provide high visible contrast between tumor and normal tissue: that it sticks to the tumor and shines. That implies it collects in the tumor and clears from normal tissue; the emission wavelength is easily distinguishable from background light; and the emission is bright enough to be accurately detected.
At the moment, only three fluorescent agents see common use for FGS, indocyanine green (ICG), 5-aminolevulinic acid hydrochloride (5-ALA), and fluorescein sodium (see table).1-3 ICG and fluorescein are vascular imaging labels, so they accumulate in places with excess blood flow, which is characteristic of growing tumors. At least in the brain, ICG appears to remain accumulated in tumors about 24 hours after administration, during the so-called second-window. 5-ALA is not a fluorophore, but an intermediate compound in the heme synthesis pathway. Tumors, with their elevated metabolism, are primed to overproduce heme through another chemical intermediate, polyporphyrin-IX (PpIX). With excess 5-ALA provided, these cells produce more PpIX, which fluoresces pink.The factors that influence contrast may vary from cell to cell and tumor to tumor. However, even with that limitation, FGS has already demonstrated its value on some of the toughest tumor targets of all.
Taking on tough problems
Cancer attacks just about every organ, but perhaps most devastating of all are the 100 or so different cancers in the body’s most valuable real estate: the brain. Some parts of tumors are visible to the naked eye, but borders are often indistinct. Unlike other parts of the body, a surgeon looking to remove a brain tumor can’t palpate to detect tumor tissue. And, also distinct from other organs, removing extra tissue comes at very high risk.
High-grade gliomas are the most aggressive of the brain tumors: hard to see, impossible to palpate, and aggressive if not resected—but removing an extra “safety margin” may lead to debilitating neurological deficits. Although preoperative CT or MRI can identify the core tumor regions, when the cranium is opened for surgery, a “brain shift” on the order of 1 cm renders that preliminary information nearly useless.
If any situation is primed for the use of FGS, this is it.
In 2006, William Stummer and his colleagues at 17 surgical centers in Germany completed the first large-scale clinical trial of FGS.2 Surgeons aided by PpIX visible light fluorescence after 5-ALA administration resected tumors about twice as efficiently as surgeons working under standard white-light illumination. The percentage of patients with progression-free disease at six months post-surgery—a standard benchmark for cancer treatments—also doubled for those undergoing FGS.
Since then, additional FGS studies with 5-ALA,2 fluorescein,3 and ICG3 have reflected similar improvements in gross total resection (GTR), a primary measure of surgical efficiency for brain tumors and other targets, such as skin, colorectal, pancreatic, and more than a dozen other cancers .3
Professor Keith Paulsen, Scientific Director of the Center for Surgical Innovation at Dartmouth-Hitchcock Health (Lebanon, NH), was part of one of the early North American groups to use 5-ALA for tumor imaging: “We had several eminent neurosurgeons observing—our lead surgeon, when he first saw it, said, ‘it looks like a volcano.’”
Getting specific
The success of approved imaging agents—in general, a doubling of gross total resection—sounds impressive, but even Stummer’s initial influential large-scale study only demonstrated a two-thirds chance that a tumor would be fully removed. It’s enough to show the value of FGS, but also enough to show that more-specific fluorescent markers are needed.
The formula for building a more-specific marker starts with finding a compound that is present only in tumors or, more realistically, mainly in tumors. Then, find (or build) a targeting moiety—an antibody or antibody-like molecule, a peptide, a small molecule, or a nanoparticle—that binds strongly to the target compound. And then, conjugate that with a fluorophore that is bright.3-6 Bonus points if the marker fluoresces in the near-infrared (near-IR; also known as NIR), where tissue absorption and scattering are minimized. It’s conceptual simplicity, but brimming with practical challenges.
Paulsen and his Dartmouth colleagues had concentrated on device development to improve surgical outcomes, and, he says, “we were frustrated that there weren’t more fluorophores coming available for use.” In the context of a National Cancer Institute (NCI) program to accelerate targeted fluorophore development, the Dartmouth team conjugated a binding protein from Affibody (Solna, Sweden) to a fluorophore from Li-Cor (Lincoln, NE) to create a novel imaging agent, ABY-029.
This 58-amino acid peptide binds to endothelial growth factor receptor (EGFR), which is highly overexpressed in tumors. With absorption at 776 nm and emission at 792 nm, the new imaging agent is 3X brighter than ICG, and has no observable side effects. ABY-029 is just completing its microdose trials. “We’ve demonstrated that ABY-029 accumulates preferentially in tumors and that we can detect fluorescence, even at very low doses,” Paulsen said. “Now we’re moving into a Phase II study, looking at the efficacy of the agent as a diagnostic, then at its effect on surgical accuracy.”
Rui Tang, an instructor in the Radiology Department at Washington University-Saint Louis (WUSTL), is working with his colleagues on a different approach to a targeted fluorophore. LS301 is an eight-amino acid peptide conjugated with the small near-IR fluorescent cyanine dye cypate.3 It absorbs around 780 nm, emits around 820 nm, and selectively binds to phosphorylated annexin A2 (pANXA2), a protein upregulated in tumor cells. LS301 has strong affinity for pANXA2, particularly in the presence of elevated calcium levels. Preclinical testing has demonstrated excellent correlation between fluorescence and tumor location.
According to Tang, one key feature of LS301 is that it targets a general mechanism for cancer expression. “If you target a specific pathway, that might be unique to a given cancer, or even the stage of development of a tumor,” he said. “As long as the cancer wants to progress it will overexpress annexin and calcium.” Another key feature is LS301’s simple chemistry, which keeps its cost low and makes its storage simple. “Our goal is to improve the standard of care even in developing countries with limited resources.”
The Dartmouth and WUSTL imaging agents are just two of dozens in various stages of development.3, 4 That’s only half of the answer, though. Visualizing fluorescence requires the right illumination and imaging systems.
Seeing straight
FGS optical systems are designed with the same goal as that of the fluorescent probes: support high-contrast imaging to discriminate tumor from normal tissue. To minimize background, illumination should be limited to a narrow band at the fluorophore excitation wavelength, but to provide reference for the surgeon, white light illumination is also optimum. Depending upon the intended use and the emission wavelength of the fluorophore, that can be achieved either through a display that overlays a white light image and narrowband image, or by alternating broadband and narrowband illumination for direct viewing.Within that context, a wide range of imaging systems are available or in development. For brain tumors, for example, surgeons typically perform these surgeries while visualizing through an operating microscope. Microscope manufacturers have developed custom modules for visualizing different fluorophores to integrate with current practice. Several companies have developed handheld modules for illuminating and imaging near-IR fluorescence. And, some groups are working on bare-bones systems incorporating off-the-shelf photographic filters for fluorescein imaging.3, 4
Suman Mondal is an instructor in the Radiology Department at WUSTL, part of the team also developing LS301. Mondal is designing imaging hardware to support FGS with near-IR fluorophores. In addition to imaging performance, their group is prioritizing two other requirements: minimizing impact in crowded operating rooms, and presenting a natural view to the surgeon. They’re addressing both those requirements with an augmented-reality head-mounted display they call the Goggle Augmented Imaging and Navigation System (GAINS).3
GAINS incorporates a near-IR illumination LED, a CMOS sensor, optical filters, head-mounted displays, and the image processing program to acquire and merge broadband and fluorescence images (see Fig. 4). They’ve been able to leverage advances in other applications. For example, Mondal says, “surveillance cameras have pushed near-IR sensor development from a quantum efficiency of 25% to 60% in just a few years.” GAINS has already demonstrated the ability to accurately visualize tumor margins in breast cancer, providing early validation of the technology. Mondal is working closely with clinicians, so their design iterations are driven by surgeons’ feedback. “About 30,000 women a year in the U.S. need repeat surgery because of incomplete resection,” he said. “Being part of a solution that will reduce the burden on the healthcare system is a big motivation for our work.”Shining a light on future developments
Evidence supporting the value of FGS is rapidly accumulating, but is it the trend of the future, or a niche application?
Zhen Cheng is an associate professor of radiology at Stanford University. He and his colleagues across several institutions recently reported on the use of multispectral FGS that may pave the way for more rapid adoption. The team removed liver tumors from patients who had earlier been injected with ICG. They acquired visible light images, NIR-I images between 700 and 900 nm, and NIR-II images covering 1 to 1.7 µm. They acquired more than 1000 IR images, about 500 in each spectral window. The NIR-II window demonstrated several advantages over NIR-I. Longer-wavelength images were not degraded when room lights were on, they showed a higher tumor-to-normal tissue intensity ratio, and were capable of detecting tumors at greater tissue depths.NIR-II images of ICG fluorescence identified tumors that had not been detected preoperatively, and NIR-II data was a critical factor in modifying the surgical strategy for several patients. Still, the ICG NIR-II fluorescence detected less than 60% of the preoperatively identified lesions. “It is crucial,” said Zheng, “to develop targeted probes to significantly improve detection rate.” He believes innovative ideas will be necessary to support the development of new targeted imaging agents, given that they don’t present a big profit center for commercial interests. Zheng’s team plans to pursue multicenter clinical trials stimulating further advances of imaging agents and imaging systems. At the moment, he said, “our data shows that FGS can really help surgeons and patients.”
REFERENCES
1. D. Y. Zhang et al., Neurosurgery, 85, 3, 312–324 (2019); doi:10.1093/neuros/nyy315.
2. M. Taniguchi and J. S. Lindsey, Photochem. Photobiol., 94, 290–327 (2018); doi:10.1111/php.12860.
3. C. M. Erdman et al., Child. Nerv. Syst. (2020); doi:10.1007/s00381-020-04857-3.
4. T. Noh et al., Neurosurg. Clin. N. Am., 32, 1, 47–54 (2021); doi:10.1016/j.nec.2020.09.003.
5. W. Stummer et al., Lancet Oncol., 7, 5, 392–401 (2006); doi:10.1016/s1470-2045(06)70665-9.
6. O. Schober et al., Mol. Imaging Oncol., 818–827 (2020).
Shortcomings of FGS
FGS already has an impressive record of success, but plenty of room for improvement remains. For example, even in the 2006 Stummer clinical trial, only 65% of the surgeries resulted in complete resection. One problem is ambiguity around the tumor edge. Tumors infiltrate the surrounding tissue, but those infiltrative fingers have a lower density of tumor cells, which generally means lower PpIX expression, on par with that of normal tissue. Also, the visible PpIX fluorescence is strongly absorbed and scattered by tissue, so the tumor border remains difficult to identify, even when fluorescently labeled. ICG, fluorescein, and 5-ALA/PpIX can also accumulate in normal tissue.3
Those complications can reduce, or even nullify, the utility of these imaging agents.3 Perhaps even more troubling is the possibility that different types of tumors, or patient-to-patient variation, can lead to variations in pharmacokinetics that vary the fluorescence.3 Practical considerations also can reduce fluorescence—pooled blood or other fluids can block both the excitation and emitted light.
Another fact to keep in mind: FGS is an adjunct to surgery, an aid to the surgeon. In the brain especially, visible fluorescence does not mean the tissue can be removed, and inconsistencies in the blood-brain barrier mean that not all regions of low fluorescence should be untouched. As Dartmouth’s Paulsen pointed out, “We’re trying to help surgeons do a much better job; help them make the very best decisions.” The task for the surgeon is to integrate information from fluorescent imaging with prior knowledge and other observations to guide the course of surgery. Although current practice is not ideal, surgeons who have adopted FGS are confident that it will be increasingly adopted in both clinical and research settings.3
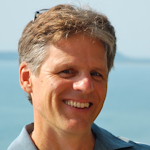
Richard Gaughan | Contributing Writer, BioOptics World
Richard Gaughan is the Owner of Mountain Optical Systems and a contributing writer for BioOptics World.