The right stuff: Lasers and optics for ultrafast microscopy
Life scientists demand higher resolution, faster image acquisition, and more choices when it comes to affordable laser options to achieve further insight into tissue and organ function. The rate of progress in this area can often be determined by the pace of developments in new commercial microscopy platforms, many of which now incorporate ultrafast laser sources for optimal in vivo imaging.
However, both ultrafast lasers and the optical components used with them have unique considerations beyond those of other microscopy techniques. Key considerations include wavelength, power, pulse duration, group delay dispersion, and laser-induced damage threshold.
Growing application space
For many years, confocal microscopy (also known as one-photon fluorescence microscopy) was among the most common optical imaging techniques for the life science community. In the past three decades, significant developments have led a shift toward multiphoton microscopy. The advantage of multiphoton microscopy over single-photon imaging is that the technique produces fluorescence signals from very small volumes, which provides high-resolution images with minimal out-of-focus light as an ultrashort-pulsed laser beam is scanned over a sample.
Ultrafast lasers are critical for many microscopy techniques, from multiphoton imaging of thick biological specimens to label-free, third-harmonic generation microscopy. The ultrashort pulse durations and high average powers of these lasers are ideal for exciting fluorophores or generating second- and third-order harmonic signals with high resolutions. Many of these lasers operate between 650 and 1064 nm and have high repetition rates, which has proven beneficial in biological, living samples. The long wavelengths result in reduced scatter and absorption that allows the multiphoton process to penetrate deeper into biological tissue, thus generating clear and high-resolution images without damage to the sample.
Choosing the right laser
There are several key parameters that should be considered when choosing the most effective ultrafast laser for two-photon microscopy setups.
Wavelength. The laser wavelengths needed are determined by the excitation wavelengths of the targeted fluorescent markers. Diode-pumped solid-state systems, such as titanium sapphire (Ti:sapphire) lasers, were the first laser systems to deliver ultrashort pulses (sub-150 fs) and broad wavelength coverage, typically ranging from 780 to 980 nm. The ability to apply wide tunability to excite several fluorescent probes has, until now, positioned Ti:sapphire lasers as the default system of choice for many imaging applications, from UV-excited fluorophores to orange and red dyes excited around one micron.
Fluorescent probes typically have large excitation bandwidths. This opens an opportunity for a range of labels to be excited by less complex and more cost-effective fixed wavelength femtosecond lasers. The first fixed-wavelength sources operated between 1040 and 1060 nm; however, a new generation of ultrafast lasers operating at 920 to 950 nm have equipped researchers with effective imaging capabilities at key wavelengths for exciting fluorescence in GCamP markers and other fluorophores.
Power. Fixed wavelength fiber lasers deliver higher average powers compared to Ti:sapphire sources, which typically average less than 2 W. The higher and more stable the average power, the more useful the source becomes to support holographic or spatial light modulator (SLM)-based imaging setups where the ultrashort-pulsed light is spread across a sample area for excitation of multiple targets (see Fig. 1).
Pulse duration. Pulse duration at the sample plane is another key consideration when choosing the right ultrafast laser for two-photon microscopy. The pulse duration at the sample plane depends on both the pulse duration coming from the laser and the properties of the subsequent materials the pulse transmits through. Advances in dispersion management have reached the stage where pulse compression optics can be engineered into the laser head of fixed wavelength fiber systems. This is ideal for managing dispersion through optical components such as microscope objective lenses.
Usability and accessibility. Researchers often face budgetary constraints and operational challenges when choosing a system with the desired laser parameters. Laser-integrated setups often come with high maintenance costs in addition to the initial equipment purchase. End-user training is usually required for these complex setups, ranging from operating-related software on a bespoke user-interface to the fundamental optical alignment of the laser. With the advent of fiber-based lasers that incorporate dispersion control, are easy to use, and robust enough to survive shipment without the need for an engineer to install the system onsite, there is a growing interest in the capabilities these laser systems can bring to individual research groups.
Choosing the right optics
The short pulse durations and high peak powers of ultrafast lasers make selecting the proper mirrors, objectives, filters, and other optical components used with them more challenging.
Chromatic dispersion is minimal for many other types of laser systems, but the short pulse durations of ultrafast sources result in a wide wavelength bandwidth (due to the Heisenberg uncertainty principle). Consequently, the pulse duration naturally broadens as these pulses pass through optical media. This lowers the peak power at the sample plane and reduces system performance. Techniques such as multiphoton microscopy depend on the laser intensity squared, so any pulse broadening will decrease the signal significantly.
In most optical media, longer wavelengths travel faster than shorter wavelengths due to group delay dispersion (GDD), which is wavelength-dependent. Most media exhibit positive dispersion when excited by two-photon sources.1 This chromatic aberration is characterized by the component’s GDD, which is defined as the derivative of the inverse group velocity with respect to frequency multiplied by optical path length. Ultrafast optics are designed to have either a GDD of zero (this would result in no pulse spreading) or a negative GDD (this would balance out the positive GDD experienced in other optical media).
Figure 2 shows how pulse-compressing mirrors with a negative GDD can be used to counteract the positive GDD of other optical media, resulting in a compressed final pulse. Dispersive prisms and gratings can be used for pulse compression instead of highly dispersive mirrors, but prisms and gratings typically suffer from more third-order dispersion, less throughput, and higher sensitivity to alignment and separation distance. Third-order dispersion results from the frequency dependence of GDD. Highly dispersive mirrors use coatings with wavelength-dependent penetration and a multi-resonance effect to provide high throughput, zero third-order dispersion, and pulse compression over a broad wavelength range.2Filters and other optics included in ultrafast microscopy systems will likely introduce some amount of positive dispersion, making it important to incorporate pulse-compressing optics. Reflective microscope objectives and beam expanders can be used to further minimize chromatic aberrations, as their all-reflective designs are wavelength-independent and therefore have negligible GDD.
Pulse stretching due to GDD is not an important concern for long pulse durations, but it impacts short pulses more significantly. Figure 3 shows the output pulse duration (τOut) for different input pulse durations (τIn) and GDD values. For example, a 10 ps pulse would experience negligible dispersion, while a 10 fs pulse would be stretched to almost 100 ps in a material with a GDD of 105 fs2.Laser-induced damage threshold
For all types of laser systems, the specified laser-induced damage threshold (LIDT) of optical components should be considered to reduce the risk of component and system failure. For pulsed lasers, LIDT specifies the maximum laser fluence the optics can withstand before damage, typically given in J/cm2. Ultrafast LIDT also comes with unique challenges because the ultrafast lasers’ incredibly short pulse durations can lead to different damage mechanisms and interactions with optical coatings and components.
Continuous-wave (CW) lasers and pulsed lasers with long pulse durations can cause damage through thermal effects and dielectric breakdown, or electrical current flowing through the component. Meanwhile, ultrafast lasers with pulse durations on the order of femtoseconds or picoseconds can cause damage through nonlinear effects such as multiphoton absorption, multiphoton ionization, and tunnel ionization.3 The prevalence of these effects increases the likelihood of damage compared to lasers with longer pulse durations at comparable powers.
When looking at the specified LIDT of optics for your ultrafast microscopy system, ideally the specification is given at a wavelength, pulse duration, and repetition rate close to that of your real system. Scaling a given LIDT specification to other use parameters is not an exact science, and commonly used scaling equations become inaccurate at low pulse durations. In general, shorter wavelengths, shorter pulse durations, and higher repetition rates all contribute to a higher likelihood of damage, and therefore a lower LIDT.
Figure 4 shows real examples of images captured using ultrafast microscopy, all of which make it possible to differentiate between different cell types.4,5Considering the key parameters of wavelength, power, pulse duration, group delay dispersion, and laser-induced damage threshold provides ultrafast microscopy system integrators with a good starting place for selecting the most suitable ultrafast lasers and optical components.
REFERENCES
1. A. Ghatak and K. Thyagarajan, Fundamentals of Photonics (2008); doi.org/10.1117/3.784938.ch7.
2. V. Pervak et al., Opt. Express, 16, 10220-10233 (2008); doi:10.1364/oe.16.010220.
3. S. S. Mao et al., Appl. Phys. A, 79, 1695-1709 (2004); doi:10.1007/s00339-004-2684-0.
4. See https://bit.ly/3q9A88L.
5. See https://bit.ly/3BUCCKs.
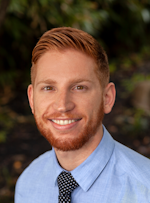
Cory Boone | Technical Marketing Manager, Edmund Optics
Cory Boone is the Technical Marketing Manager at Edmund Optics’ Barrington, NJ, USA office. He is responsible for managing the creation of technical marketing content, including application notes, published articles, web copy, email campaigns, video scripts, trade show materials, case studies, and other marketing literature. He plans the company’s technical content strategy and acts as the technical voice of the marketing department. Cory is also active in educational outreach and spreading general awareness of the optics industry. He received a B.S. in Optical Sciences and Engineering along with minors in Math and Material Science Engineering from the University of Arizona.
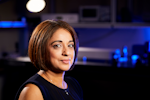
Shahida Imani | CEO, Chromacity Ltd
Shahida Imani was appointed as CEO of Chromacity in August 2018. A Chartered Management Accountant, Shahida has worked for number of high-tech companies such as Optos, Voxar, and Odos Imaging, bringing expertise in scale-up and international expansion.