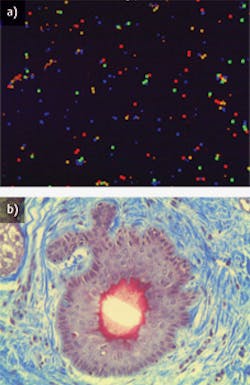
In optical microscopes used in biomedical imaging, objectives are bio-optics workhorses. They gather the light from the specimen under observation, then focus the collected light to produce a magnified, real image. Objectives also determine magnification and resolution that allow observation of fine detail.1
"In general, the objective is the most important part of the microscope—and life scientists need to know there are lenses specifically tailored for their type of science," states Stephen T. Ross, Ph.D., general manager, Product and Marketing Department, Nikon Instruments (Melville, NY). In microscopes, objectives have a multi-element design—typically containing several optical elements like lenses and mirrors to refract or reflect the light necessary for imaging. Available in magnifications ranging from 2X to 250X, objectives are available in two main categories—refractive and reflective—and it is important to know the differences between the two to suit specific imaging requirements. "Whether it's taking into account the probes and dyes needed for fluorescent microscopy, to selecting the immersion lens that best matches your research with an aqueous solution, or even just understanding the cover glass and working distance requirements of the model system you are working with, matching your objective to your application will better your chances for success," he adds.
Learning the basics
While objectives manufacturers have designed features to meet particular requirements for many applications, the issue of refractive vs. reflective is arguably the most important. Refractive objectives, which are used primarily in microscopy applications that require high, detailed resolution, are the most common. Their optical elements refract—or bend—the light that passes through the microscope, and the elements are often antireflection-coated to reduce reflections and boost light throughput.2 Typically, they must use a cover glass, alternately known as a cover slip, over the specimen to protect it, as refractive objectives have a narrow depth of field.
By contrast, reflective objectives use mirrors to magnify and reflect light to produce images. Unlike refractive objectives, reflective objectives reflect light using metallic surfaces rather than glass, thereby avoiding the aberrations (imperfections in image formation) that can be present when using refractive designs. What's more, reflective objectives enable working deeper in the ultraviolet (UV) and infrared (IR) spectral regions because of their ability to overcome aberrations. They are corrected for direct sample observation, with no need for a cover slip between the sample and objective.
"Life science microscopy applications today have incorporated the best features of both categories into one highly functional workstation," explains Gary Czarnecki, National Finescope Technical Supervisor at Mitutoyo America (Aurora, IL). Such microscopy applications include confocal laser scanning, fluorescence resonance energy transfer (FRET), and near-infrared (NIR) live cell imaging, which routinely blur the division between refractive and reflective, he says. To that end, researchers can now perform multiple techniques on a single instrument that in the past may have required several separate microscopes.
Life science utility
"There are two popular uses for objectives in life sciences today—high magnification biomedical imaging and laser focusing or tracking," explains Stephan Briggs, biomedical engineer at Edmund Optics (Barrington, NJ). The life sciences work that the company deals with primarily utilizes infinity-corrected objectives for fluorescence and confocal microscopy (see Fig. 1). Infinity-corrected objectives, which literally focus at infinity, allow for optical components such as filters, beamsplitters, and polarizers to be utilized in the optical path without inducing any chromatic or spherical aberration, he explains.
For life scientists working on brain imaging, for example, a lens tailored to provide the highest aperture (the opening that determines the cone angle of a bundle of rays that come to a focus in the image plane) at the greatest distance while correcting for spherical aberration that occurs deep into the tissue is paramount, explains Ross. Recognizing this, Nikon provides oil and water immersion (OI and WI) lenses with correction collars to compensate for spherical aberration, allowing scientists to look millimeters into the brain, and still get optimal resolution and sharp contrast, he adds.
"Live cells used in imaging, which are primarily water, will limit the numerical aperture (NA), and have the best correction for spherical aberration when the lens immersion media is index-matched to the live cells," continues Ross. So Nikon now offers two WI objectives—the 40X WI and 60X WI IR Lambda S series—that offer 1.25 and 1.27 NAs, respectively. Previously the limit was 1.20 NA, he says.
Mitutoyo America manufactures a variety of 95 mm infinity-corrected objectives. When used with the company's MF-U two- and three-axis measuring microscopes, they are well suited to microscopy applications that require very long working distances (>2 mm) between sample and objective, explains Czarnecki. For example, their MF-U microscopes can examine specimens at a magnification of up to 4000X with a working distance of 13 mm using their Standard M Plan Apochromat 200X objective and 20X eyepieces. The company's FS70 Series microscopes are available in three optical body configurations for brightfield, yttrium aluminum garnet (YAG), and UV laser systems: the FS70L-S and FSL4-S models work with YAG and UV laser systems, respectively, and, when used in combination with brightfield, NIR, near-ultraviolet (NUV), and UV objectives, can easily adapt to life science applications (see Fig. 2).What's trending
"Over the last decade, the shift to live cell examinations has resulted in improved NIR/NUV microscope objectives capable of viewing green fluorescent protein (GFP)-labeled cell structures over a wide range of new dye frequencies. This trend continues today," says Czarnecki. Specifically, he adds, NIR illumination/objectives coupled with new red GFP dyes has shown to be an excellent method to view cell surface structure and internal chemistry through various new GFP analog markers.
Ross agrees, saying that red to NIR light penetrates deeper into live tissue and is friendlier to live cells. So Nikon is tuning its offerings to meet this trend, without sacrificing transmission in the visible range, through use of a new coating technology: Nano Crystal Coat employs multiple layers of extra-low refractive index material to enable high transmission at very high apertures.
REFERENCES
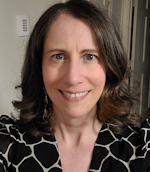
Lee Dubay | Managing Editor
Lee Dubay is managing editor for Laser Focus World. She is a seasoned editor and content manager with 20 years of experience in B2B media. She specializes in digital/print content management, as well as website analytics, SEO, and social media engagement best practices.