OPTICAL COHERENCE TOMOGRAPHY/OPHTHALMOLOGY: Intraoperative OCT improves ophthalmic surgery
EDITOR'S NOTE: This article is updated and adapted from S. Binder, Spektrum der Augenheilkunde, 28, 1, 2–5 (2014), with permission from Springer.
The most popular examination method in ophthalmology today is optical coherence tomography (OCT). Traditionally, OCT examinations have been performed pre- and post-operatively in vitreo-retinal surgery. While this application of the technology has enabled outcomes to be measured and results to be documented, surgeons have not had the ability to incorporate the results to improve surgical treatments. But with considerable advances to OCT—including high-definition OCT (HD OCT), 3D visualization, and adaptive optics—the integration of these methods into the surgical microscope is logical.
The technology is now helping ophthalmologists carry out delicate eye surgeries. In today's integrated microscopy systems, OCT uses the optical pathway of the microscope, giving the physician seamless and simultaneous control of both surgical manipulations and OCT visualization. The result is enhanced information and control for the surgeon, and improved outcomes for the patient.
This article is an overview of the currently available commercial devices available for intraoperative OCT imaging. Those I have used personally are described in more detail.Envisu SDOIS by Bioptigen
Bioptigen's Envisu spectral-domain ophthalmic imaging system (SDOIS) is a spectral-domain OCT (SD-OCT) system designed to acquire, process, display, and save depth-resolved images of ocular tissue microstructure (see Fig. 1). It is primarily intended for the imaging of retinal tissue: It enables researchers to resolve in fine detail the microstructure of the retina—such as the nerve fiber layer, the inner and outer plexiform, and nuclear layers—and those of the retinal pigment epithelium, as well as the vessels of the choroid. But by simply changing the focal position, it is also possible to image the cornea, sclera, and conjunctiva with no increase in optical power. Indications for clinical use include the evaluation of ophthalmic tissue in routine clinical examinations and as an aid in the diagnosis of conditions that affect the optical scattering properties of ocular tissue (see photo at top of this page).
This sophisticated OCT hardware and software system enables real-time noninvasive imaging of internal tissue microstructure, extending the SD-OCT application space to include imaging of patients throughout the clinical environment and imaging a wide range of animal subjects. It is ideally suited to image the retina in small animals (rodents, rabbits), large animals (such as dogs and pigs), and humans.
The SDOIS, which received FDA clearance 510(k) in 2006, includes a handheld probe and a microscope setup. The axial resolution is 5 μm and A-scan acquisition rate is 27 kHz.1 The device was adapted on a binocular indirect ophthalmolo microscope (BIOM).
iVue by Optovue
Optovue's SDOCT-based iVue offers complete retina, glaucoma (including ganglion cell complex analysis), and anterior segment scanning. It received clearance by the FDA through a 510(k) procedure in 2009. Axial resolution is 5 μm; A-scan acquisition rate is 27 kHz.
OCT camera by OPmedT
OptoMedical Technologies GMBH (OPmedT; Lübeck, Germany) has developed an OCT camera that can be mounted on a surgical microscope, the Hi-R 900 by Möller-Wedel GmbH. The camera can be used to visualize the anterior and posterior segment of the eye, and OPmedT indicates the following surgical applications: corneal transplantation (including lamellar keratoplasties), glaucoma surgery, cataract surgery, and retinal surgery (e.g., ILM peeling).
3D-OCT 2000 by Topcon
Topcon's 3D-OCT 2000 incorporates SD-OCT and a high-resolution fundus camera. Axial resolution is 5 μm; A-scan acquisition rate is 27 kHz.
SOCT Copernicus by Optopol
Optopol's SOCT Copernicus includes progression analysis software that calculates disc damage likelihood, symmetry between the disc, and retinal nerve fiber layer (RNFL) thickness. Axial resolution is 6 μm; A-scan acquisition rate is 27 kHz.
Spectral OCT SLO by Optko
Optko's device combines SD-OCT, scanning laser ophthalmology (SLO), and microperimetry. Axial resolution is 6 μm; A-scan acquisition rate is 27 kHz.
Spectralis OCT by Heidelberg Engineering
Heidelberg Engineering's Spectralis includes a high-speed SD-OCT system with eye tracking, fluorescein angiography, ICG angiography, and autofluorescence. Axial resolution is 4 μm; A-scan acquisition rate is 40 kHz.
Cirrus HD OCT and OPMI VISU 200 by Carl Zeiss Meditec
Carl Zeiss Meditec's Cirrus HD-OCT system connects directly to the Zeiss OPMI VISU 200 BrightFlex surgical microscope, and uses the microscope's optical pathway. My tests of the system involved producing mostly 512 Å~ 128 macular cube scans during various steps of microsurgical procedures. My group evaluated B-mode scans for quality immediately, and repeated the scans if necessary. A BIOM 60 D lens or corneal lens aided in-depth visualization during posterior segment surgery. We post-processed and visualized the acquired volume data with a ray-traced 3D display system.2
The value of a microscope-integrated system—as compared to a microscope-mounted system—became clear when attempting precise measurements of the foveal thickness in eyes undergoing uneventful cataract surgery.3 In fact, our group has used this system for several indications in macular surgery, retinal detachment surgery, and cataract surgery in hundreds of cases. Comparing the use of dye for imaging epimacular tissue and the use of simultaneous OCT visualization during membrane peeling, use of the OCT technique (which requires no preparation) proved nearly as informative.4 In addition, 3D post-processing of the cubes enabled access to more complex information about tissue behavior during the surgical process, which proved useful for scientific use and teaching.
The corticosteroid Triamcinolon (Volon 40) was found to be useful not only for enhancing the reflectivity of tissue visualized with routine OCT, but also for visualizing in greater depth tissue that is otherwise invisible with routine OCT because of low density.5
The axial resolution of the Cirrus HD OCT is 5 μm; A-scan acquisition rate is 27 kHz. Both instruments are CE-approved.
In using this setup, we discovered some limitations. Primarily, it was problematic for the surgeon to shift focus to another screen to see the OCT images. Also, it was difficult to localize small tissue remnants because the instrumentation offers no tracking function. The surgeon could sharpen the OCT picture using a screw on the microscope, but had otherwise no direct control over the system. An assistant was needed in the OR to trigger the scan, which could lead to some time delay during the surgery. Cables between the OCT machine and the microscope for data acquisition and OCT were hindering during surgery and between cleaning, that made the device more vulnerable. All these experiences led to the development of a new system, Zeiss's OPMI Lumera 700/Rescan 700.
OPMI Lumera 700/Rescan 700 by Carl Zeiss Meditec
The OPMI Lumera 700/Rescan 700, released in 2014, is the first commercially available, fully microscope-integrated, continuous HD OCT instrument (see Fig. 2). It uses the microscope's optical pathway for real-time imaging. This CE-certified system, which is also FDA-approved as of November 2014 and was developed for ophthalmic use only, provides simultaneous OCT imaging for anterior and posterior segment surgery. The OCT instrument is integrated without cables into the footstand of the Lumera 700 microscope and the HD OCT camera integrated in the microscope's head without losing any other function of the surgical microscope. Both functions have an electronic interface for remote control via a support system (Zeiss's Callisto).The OCT camera can be controlled using either the microscope's foot pedal or the sterile touchscreen interface. During surgery, the surgeon also has complete control over all OCT functions—including start and stop of scan, positioning of the scan, recording of line and cube scans, tracking of scans, and review—from the foot pedal. Alternately, these functions can be controlled using the touchscreen if data acquisition is also performed. A vertical and horizontal OCT picture is mirrored into the side and on the top of the surgeon's right ocular.
Both the standard surgical video and simultaneous OCT video are visible on the screen. The device can be used unaided for anterior segment surgery to measure corneal thickness, anterior chamber depth, iris thickness, and chamber angle. It is suitable for observing and controlling steps in cataract surgery, including corneal/limbal incisions, capsulorhexis, and dynamics of phacoemulsification. Furthermore, the system can detect residual viscoelastic and control the position of the lens implant during and at the end of surgery.
For posterior segment surgery, either a corneal lens or a wide angle viewing system (Resight) is necessary to gain high-quality pictures. During surgery, pre-retinal vitreous mobility and adhesions, the presence or absence of an attached posterior hyaloid, pre-retinal membranes, or foveal destructions (holes, thinning, edema or iNLOnl-loss, sub-retinal fluid, neo-vascularization, or hemorrhage) can be continuously visualized. Tearing forces applied to the optic nerve and retina can be detected, controlled, and minimized. Scans of sufficient quality can also be acquired under gas, perfluorocarbon (PFCL), or silicone instillation.
A progression
In addition to these currently available commercial systems, others are being developed. Bioptigen recently received a $1.72 million grant from the U.S. National Eye Institute (NEI; Bethesda, MD) for development of microscope-integrated intraoperative OCT technology. In September 2014, Hiroko Terasaki of Nagoya University Hospital (Japan) presented prototype intraoperative endoscopic OCT from NIDEK at the Meeting of Club Jules-Gonin. Professor Terasaki and her group have used the handheld forward-imaging, 21-gauge (820 μm diameter) needle endoscope for ophthalmic OCT inspection of cornea, lens, and vitreous removed from ex vivo porcine eyes. The device proved able to clearly image structures 2.5 mm from the probe tip, meaning that it proved suitable for endoscopic inspections. The idea is similar to work done by Han et al.,6 but uses a 23G fiber.
All of these systems are examples of a major shift in the application of OCT for surgical guidance. Moving forward, microscope-integrated examination systems will surely be used in ophthalmic microsurgery with increasing frequency.
Integration of OCT in surgical microscopy adds considerable value without loss of time or sterility. Use of the microscope's optical pathway and inclusion of OCT imagery in the surgeon's view enables complete and seamless control. This is a fabulous example of how new technology can address familiar challenges. And, as is often the case with new technology, further experience might enable detection of yet-unrecognized processes—and thus yield even greater results.
DISCLOSURE
The Ludwig Boltzmann Institute (LBI) for Retinology and Biomicroscopic Lasersurgery (LBI) received an unrestricted equipment grant by Carl Zeiss Meditec. LBI received a research grant by Carl Zeiss Meditec in 2011/2012. The author declares no actual or potential conflicts of interest in relation to this article.
REFERENCES
1. Y. K. Tao, J. Ehlers, C. A. Toth, and J. A. Izatt, Opt. Lett., 35, 3315–3317 (2010).
2. S. Binder, C. I. Falkner-Radler, C. I. Hauger, H. Matz, and C. Glittenberg, Retina, e-pub ahead of print (Jan. 26, 2011).
3. Zeiler et al., Acta Ophthalmol. Scan. (2014); in print.
4. Falkner et al., Ophthalmology (2014); in print.
5. M. Esmaeelpour et al., Invest. Ophthalmol. Vis. Sci., 22, 55, 8, 5074–5080 (2014); doi:10.1167/iovs.14-14646.
6. S. Han, M. V. Saunuic, J. Wu, M. Humayun, and C. Yang, J. Biomed. Opt., 13, 020505-1–020505-3 (2008).
About the Author
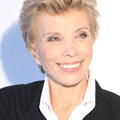
Susanne Binder, MD
Professor and Chair of the Department of Ophthalmology
Susanne Binder, MD is Professor and Chair of the Department of Ophthalmology at The Ludwig Boltzmann Institute for Retinology and Biomicroscopic Laser Surgery, Rudolf Foundation Clinic (Vienna, Austria), and Adjunct Professor of Ophthalmology, Cornell University (New York, NY). She has authored four books and numerous articles and journal papers, including Intra-operative OCT devices for ophthalmic use: an overview (Spektrum der Augenheilkunde 28, 1, 2-5; 2014). She has been using OCT in surgery since 2008.