SPECTRAL IMAGING: Real-time spectral unmixing enhances understanding of cellular biomarkers
Microscopic imaging of cellular sample morphology has been used to diagnose medical disorders for centuries. Recent years have brought the discovery of numerous molecular biomarkers for various diseases—and now, simple chemical assays are playing an increasing role in primary diagnosis and determination of medical treatment. But because individual biomarkers generally lack the sensitivity and specificity to completely identify a disease state, researchers yearn for the simultaneous use of multiple biomarkers.
Combining multiple molecular biomarkers with traditional microscopy would mean that biomarkers could be used both to locate potential abnormal regions on a microscope slide and to clarify the interpretation of those abnormalities. But coupling biomarkers to stains to make them easily recognizable often confuses things, because the colors become hard to interpret by eye.
Analyzing such complex microscope slides with multispectral imaging, however, provides a detailed spectrum for every pixel in the image. This article describes a multispectral system optimized for fast and efficient analysis of traditionally stained clinical pathology samples to which molecular labels have been added. The instrument can clearly display results in real time as the magnified image is scanned across a large slide.
Multispectral brightfield microscopy
Multispectral imaging systems provide detailed spectral information for every pixel. If the pixels are imagined as arranged in a matrix in the x-y plane, the information can be displayed along the z-axis in a 3D data structure called an "image cube." Linear spectral unmixing is a spectral imaging analysis technique: It assumes that the total acquired spectra at each pixel can be expressed as a linear combination of contributions from a series of independent elements, each having its own distinct "reference spectrum." The unmixing technique allows the final image to be decomposed into separate "rule images," one for each of the reference spectra. The intensity of each rule image corresponds to the calculated amount of that spectral component present at each pixel. If the image of a microscope slide contains overlapping spectra from multiple biomarkers that are difficult to distinguish visually, multispectral imaging makes it possible to analyze the image and spectrally unmix the reference spectra of each individual biomarker to cleanly separate the different molecular components.
Cellular morphology and tissue architecture are conventionally identified using colored absorptive transmission stains such as hematoxylin, eosin, Papanicolauo stain (Pap stain), and Masson's trichrome. In contrast, molecular biomarkers can be labeled with either fluorescence probes or transmission stains. Fluorescence labeling generally has the advantage that the spectrum of emission can be quite narrow, and for situations requiring simultaneous tagging of multiple biomarkers, it is possible to choose labels with minimal spectral overlap that are clearly distinguishable—either by eye or with a proper set of interference filters.
Including both fluorescent labels and traditional transmission morphology stains on a single slide makes labeling problematic, though. Hematoxylin and other common stains contain an aluminum "mordant" that quenches fluorescence, making it difficult to obtain adequate signal from fluorescently labeled biomarkers in their presence. In addition, most commonly used transmission stains both absorb and fluoresce strongly themselves throughout the visible wavelength region. Concentrations of these stains vary throughout the sample—a fact that produces two results: a spatially varying decrease in biomarker fluorophore excitation and emission efficiency (due to the stain absorbance), and spatial variations in background fluorescence (due to the stain's own fluorescence). This breaks down the linearity of the spectral addition of each component, and makes linear spectral unmixing invalid.
Thus, multispectral imaging of biomarkers, multiplexed with morphology staining, is significantly more accurate when accomplished using transmission rather than fluorescent biomarker staining.1 Fortunately, many transmission stains are stable and interact minimally with other stains. But transmission stains typically have broad absorbance profiles, so when even just a few of these combine with morphology, results often cannot be interpreted clearly by eye. Multispectral imaging can, however, separate the morphology staining components from those of the various biomarkers even when the stains in question are highly overlapping both spectrally and spatially.
The number of wavelengths needed for accurate spectral imaging depends on the number of stains used, the degree to which they spectrally overlap, and the complexity of the sample being imaged. Figure 1 illustrates this important point. Pap staining of cervical cells is the traditional diagnostic approach to cervical cancer detection. On the far left (a) is a color image of Pap-stained (hematoxylin, eosin, Fast Green, and OG6) cervical cells co-stained for the biomarker p16 with an additional stain, Deep Space Black. There are a total of five stains in the sample, each staining different parts of different types of cells. The unmixed hematoxylin rule image (hematoxylin is the main nuclear stain and of primary importance for morphologic evaluation) for varying number of unmixing wavelengths, N, is shown in the four images to the right (b), with a graph of relative spectral quality shown in (c), and the spectral signatures of the different stains used in the unmixing is shown in (d). For each N, the wavelengths used for the unmixing were chosen to provide the best result possible for this choice of wavelength number. Despite this image containing only five stains, the results using even the best choice of six wavelengths is far from adequate. In fact, achieving the best results requires tens of wavelengths.
Real-time multispectral microscopy
Accurately separating the signatures of multiple molecular biomarkers, combined with traditional microscopic imaging of morphology, requires spectral imaging with up to 30 wavelengths. The ideal system combines a high-speed digital camera with a filter that selects wavelengths in order and outpaces the camera frame rate in switching between them. For 30 or more colors, filtering the image with a mechanically rotating filter wheel is not practical. And while liquid-crystal tunable filters (LCTFs) can produce 30 wavelengths, switching takes a large fraction of a second. Acousto-optic tunable filters (AOTFs), however, have electronically programmable spectral flexibility and switching time of <1 ms. We have developed an AOTF-based spectral imaging system that can both image, spectrally unmix the components, and display the results on a computer screen in real time.
Figure 2 illustrates the ability of this system to image regular cellular staining and to provide identification using a molecular biomarker. In traditional Pap staining of cervical cells as shown in Fig. 2a, a typical sample contains a wide range of cell shapes and types. Human papilloma virus (HPV) is a well-known biomarker precursor for cancer. We have therefore combined HPV imaging with Pap staining, and Fig. 2b shows how the presence of HPV can be added with a red "false color" overlay to the Pap image-clearly indicating which cells have abnormal DNA.
A spectral unmixing system can also help clarify the morphology of cells by allowing separation and accurate intensity adjustment of critical components. Figure 3 demonstrates this on two images of dense clumps of cells. The full image with intense and overlapping stains is opaque, but the control provided by a spectral imaging system allows isolation of the most important hematoxylin stain and reduction of the intensity so interior features can be fully resolved.
Applications benefit
With the help of AOTFs, images produced by spectral unmixing can be used in conjunction with cell-by-cell biomarker images to produce false-colored overlays with significant additional information to facilitate more accurate interpretation.
By combining spectral information with an image capable of conveying morphology, the potential for this technique is being explored in applications such as cancer detection,2 study of neuronal action potentials,3 blood oxygenation measurement,4 and more. And in combination with other techniques such as surface-enhanced Raman scattering (SERS), it promises even greater outcomes.
REFERENCES
1. E. S. Wachman et al., J. Biomed. Opt., 19, 5, 056006 (2014).
2. R. M. Levenson et al., Expert Opin. Med. Diagn., 2, 1067 (2008).
3. Li Q. et al., Appl. Opt., 52, 17, 3891–3901 (2013).
4. R. D. Shonat et al., Biophys. J., 73, 1223 (1997).
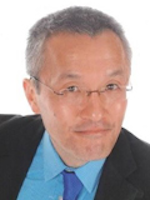
Alexandre Fong | Director, Hyperspectral Imaging
Mr. Fong is Director, Hyperspectral Imaging at HinaLea Imaging. He was previously Senior Vice-President , Life Sciences and Instrumentation and Business Development at Gooch & Housego and held technical and commercial leadership positions at ITT Cannon, Newport Corporation, Honeywell and Teledyne Optech. He holds undergraduate and graduate degrees in Experimental Physics from York University in Toronto, Canada, an MBA from the University of Florida and is a Chartered Engineer. Mr. Fong is a published author and lecturer in the fields of precision light measurement, life sciences imaging, remote sensing, applied optics and lasers. He has served on the board of the OSA as well as Chair of the Public Policy Committee, Chair of the OSA Industry Development Associates and Contributing Editor to Optics and Photonics News, he is also an active member of SPIE as a former member of the Financial Advisory Committee and the International Commission on Illumination (CIE). Alex is the current president of the Florida Photonics Cluster.
Murray Reed | Chief Technology Officer, Gooch & Housego
Murray Reed is chief technology officer at Gooch & Housego.
Elliot Wachman | VP of Research and Development, Gooch & Housego
Elliot Wachman is VP of research and development at Gooch & Housego.
Austin Dowd | Product Manager, Gooch & Housego
Austin Dowd is a product manager at Gooch & Housego.