Inexpensive white-light microscopy technique quantitatively captures both intensity and phase
Many living cells absorb very little visible light, resulting in very low-contrast images when viewed under a microscope. However, such cells still affect the phase of the light passing through them, and as a result phase-contrast microscopy can be used to turn these differences in phase into intensity changes. Phase-contrast microscopy has traditionally come in two forms: simple, low cost, and qualitative; or elaborate, expensive, and quantitative. Now, scientists at the King Abdullah University of Science and Technology (KAUST; Thuwal, Saudi Arabia) have come up with a white-light wavefront-sensing technique that leads to a simple but quantitative form of phase microscopy.
The method, which can be added to a standard commercial microscope with only small modifications, is an improvement on a speckle-tracking technique that the researchers previously developed, and uses a nonlinear model based on a generalization of the so-called transport of intensity equation (TIE), for which intensity images are taken at multiple defocused planes and the phase shifts numerically reconstructed. The microscope modifications consist of replacing the instrument’s camera with a high-resolution coded wavefront sensor consisting of a random phase mask placed 1.5 mm away from an intensity sensor, and modifying the microscope’s transillumination module for collimated but temporally incoherent light (white light). Because the illumination is collimated, the reference image is a diffraction pattern of the random phase mask—introducing a phase object distorts the wavefront in a way that is measurable by tracking movements in the speckle pattern introduced by the object. The technique allows simultaneous quantitative intensity and phase reconstruction. Reference: C. Wang et al., Sci. Rep. (2019); https://doi.org/10.1038/s41598-019-50264-3.
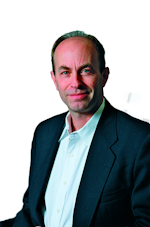
John Wallace | Senior Technical Editor (1998-2022)
John Wallace was with Laser Focus World for nearly 25 years, retiring in late June 2022. He obtained a bachelor's degree in mechanical engineering and physics at Rutgers University and a master's in optical engineering at the University of Rochester. Before becoming an editor, John worked as an engineer at RCA, Exxon, Eastman Kodak, and GCA Corporation.