Superresolution optical-fluctuation imaging microscopy is multicolor
Multicolor fluorescence microscopy enables biologists to study the relative arrangements of cellular structures, as well as interactions of different proteins. While conventional microscopes can only resolve details on the order of the wavelength of light, numerous superresolution microscopy concepts have been developed in the past decade to help researchers to overcome the diffraction limit and to make new discoveries. These new methods are only slowly finding their way into routine biological applications. This is sometimes due to the increased complexity of superresolution microscopy hardware, and sometimes due to increased demands on sample preparation and fluorescent labels. The requirements for successful superresolution imaging are even more challenging to meet for multicolor applications.
Researchers at the Laboratory of Biomedical Optics at the École polytechnique fédérale de Lausanne (EPFL; Lausanne, Switzerland) are working on superresolution optical-fluctuation imaging (SOFI) to increase the spatial resolution and sampling in 2D and 3D imaging. SOFI is an alternative to single-molecule localization microscopy techniques such as stochastic optical reconstruction microscopy (STORM) and photoactivated localization microscopy (PALM). It analyzes higher-order spatiotemporal statistics of a time series of blinking fluorophores, and as a result does not require the isolation of individual fluorophores′ emissions. SOFI is compatible with a wider range of labeling and imaging conditions, which simplifies fluorophore selection and experiments.
In their new study, the EPFL researchers, led by Theo Lasser and Aleksandra Radenovic, extended the statistical analysis into the spectral domain to pave the way towards a new approach for multicolor superresolution imaging (see figure). The number of colors is not limited by the microscope’s spectral channels.
Additional color channels
In classical multicolor imaging, crosstalk between different spectral channels of the microscope is avoided as much as possible. In contrast, in multicolor SOFI, the researchers exploit the crosstalk for generating additional color channels. They apply so-called “cross-cumulant analysis” between multiple simultaneously acquired spectral channels. The statistical analysis allows them to supplement the physical detection channels provided by the microscope with additional virtual spectral channels.
“Only the signals that are spatially and temporally correlated in the different spectral channels will appear in the virtual channels,” says Kristin Grussmayer, one of the researchers. “We are picking up exactly the crosstalk that everyone else wants to get rid of.” The additional computationally generated spectral channels, together with linear unmixing, allow the imaging of more distinct fluorophore colors than recorded physical detection channels.
The researchers have developed a framework to optimize the spectral channels of the microscope for a given combination of fluorophores that should be imaged. Simulated datasets helped the team to verify that their new multicolor approach should work for a wide range of labels with different photophysical properties, even for those with strongly overlapping emission spectra. “We could show that our approach works for three-color imaging in fixed and in living cells for a variety of dyes and fluorescent proteins,” says Grussmayer. “The imaging can be performed using commercial widefield setups with two-channel image splitting units that are widely available. In principle, we are not limited to three colors.”
Instructions for performing multicolor spectral cross-cumulant SOFI analysis are available on the Laboratory of Nanoscale biology website at https://bit.ly/SOFIPackages and the software package can be downloaded at https://bit.ly/SOFIDownload.
REFERENCE
1. K. S. Grussmayer et al., Nat. Commun. (Jun. 15, 2020); doi:10.1038/s41467-020-16841-1.
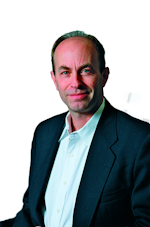
John Wallace | Senior Technical Editor (1998-2022)
John Wallace was with Laser Focus World for nearly 25 years, retiring in late June 2022. He obtained a bachelor's degree in mechanical engineering and physics at Rutgers University and a master's in optical engineering at the University of Rochester. Before becoming an editor, John worked as an engineer at RCA, Exxon, Eastman Kodak, and GCA Corporation.