PHOTODETECTORS: InP-based quantum-cascade detectors target the mid-IR
With wide-ranging potential applications in sensing, security, and defense, semiconductor-based mid-IR detector types include quantum-well IR photodetectors (QWIPs), photovoltaic QWIPs, mercury cadmium telluride detectors, and silicon microbolometers. While each of these have unique advantages in terms of speed and signal-to-noise ratio as a function of operating temperature, scientists at the University of Neuchâtel (Neuchâtel, Switzerland) have developed two narrowband indium phosphide (InP)-based quantum-cascade detectors (QCDs) operating at 5.3 and 9 µm that profit from increased absorption efficiency due to the smaller effective mass in indium gallium arsenide/indium aluminum arsenide (InGaAs/InAlAs) compared to GaAs/AlGaAs quantum-well structures.1 (Under most conditions, electrons and holes in a crystal respond to electric and magnetic fields almost as if they were free particles in a vacuum, but with a different mass-the effective mass.)
In addition to improved absorption efficiency, QCDs (like photovoltaic QWIPs) do not require a bias voltage for operation, meaning there is no dark-current noise and no integration-time limitation due to capacitance saturation in the readout circuit. Also, thanks to the lighter effective mass, the wells in a QCD can be grown somewhat wider than in a QWIP, causing less linewidth broadening due to interfacial roughness and a lower noise figure.
Quantum stair
The QCDs developed by the researchers are based on a vertical transition between two bound states in a silicon (Si)-doped quantum well, with an excited state that is coupled to the uppermost state of a chirped superlattice and forming a “quantum stair” that allows extraction of the electrons from the upper state to the ground state of the following period. In the 5.3 µm detector, for example, electrons are excited from the ground state to upper states called 8 and 9 (with transition energies of 230 and 237 meV, respectively). From these coupled states, they either jump back to the starting point or to state 7, where a series of transitions back to the ground state of the next period occurs (with subsequent transition energies of 34 meV for each state), resulting in fast vertical displacement of electrons.
Molecular-beam epitaxy (MBE) was used to grow these stair structures on an InP substrate. For the 5.3 and 9 µm devices, current-voltage (I-V) curves were derived at different temperatures. Unfortunately, their somewhat lower resistance at elevated device temperatures causes more Johnson noise, resulting in a reduced detectivity; this drawback will be corrected in future experiments by using slightly thicker barriers.
Fourier-transform infrared spectrometer (FTIR) measurements revealed the absorption and responsivity spectrum of the detectors. Peak responsivity values in the background-limited infrared photodetector regime of 3.2 and 9.0 mA/W with 4.3% and 7.1% relative linewidth were determined for the 5.3 and 9 µm detector, respectively (see figure).
In future work, the Neuchâtel research team plans to optimize the barrier thicknesses and dopant concentration of their quantum-cascade devices. Coupled with an enhanced optical diffraction grating to capture more light, performance improvements should be significant. “We hope that these detectors will reach performance levels which are sufficiently high to make them an interesting alternative to commercially available devices,” says project manager Daniel Hofstetter. “In order to fully profit from the rich physics and chemistry affiliated with this part of the electromagnetic spectrum, IR technology is still in need of reliable sources and detectors.”
REFERENCE
1. M. Graf et al., Applied Phys. Lett. 88, 241118-1 (2006).
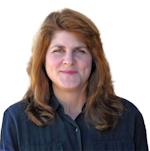
Gail Overton | Senior Editor (2004-2020)
Gail has more than 30 years of engineering, marketing, product management, and editorial experience in the photonics and optical communications industry. Before joining the staff at Laser Focus World in 2004, she held many product management and product marketing roles in the fiber-optics industry, most notably at Hughes (El Segundo, CA), GTE Labs (Waltham, MA), Corning (Corning, NY), Photon Kinetics (Beaverton, OR), and Newport Corporation (Irvine, CA). During her marketing career, Gail published articles in WDM Solutions and Sensors magazine and traveled internationally to conduct product and sales training. Gail received her BS degree in physics, with an emphasis in optics, from San Diego State University in San Diego, CA in May 1986.