Future Optics: Big optical science opens up the universe at all scales
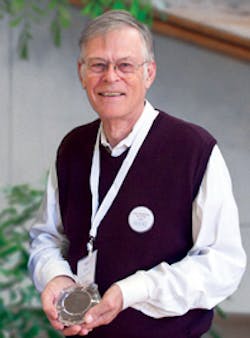
OSA: How did you get involved in optics?
Robert Byer: My grandfather was a machinist at the Mount Wilson Observatory's machine shop, and he took me to visit the 100 in. telescope as a small boy. I built my own telescopes and photographed Sputnik when it flew over in 1957. I wanted to be an astronomer, but Caltech astronomer Horace Babcock told me I should study physics first, and then go into astronomy.
As a freshman at Berkeley, I asked Sumner P. Davis if I could work with him in his laboratory. His reply was, "Go read this book on grating spectroscopy (by George Harrison) and when you understand everything in it, come back and see me." It took me all of my freshman year, but I succeeded and I worked with him for four years.
On my graduation day, he suggested I visit Spectra-Physics because "they do optical things." Nobody greeted me when I arrived, so I waited—but after 15 minutes I heard cheering and went to the back, where Earl Bell invited me in. He had just invented the mercury laser—the first visible ion laser. I was intrigued and became their 13th employee.OSA: How did you get involved with OSA?
RB: In my freshman year, OSA offered a great deal—students paid $10 a year and got Physics Today and Applied Optics for free. So I joined, and OSA became my home society. When I went to Stanford, I helped organize the local OSA chapter and, with the help of my mentor Tony Siegman and thesis advisor Steve Harris, started attending OSA annual meetings and giving talks. I found that if you do good work, pretty soon you get to know a lot of people in your home society. I met Europeans working in the field, and they became friendly competition. As a young boy in Pasadena, I had never believed that I ever would travel to Europe. Now, we do our collaborations internationally and don't give it a second thought.
OSA: Speaking of large projects, how did you get involved in the LIGO project?
RB: In 1988, I introduced the LIGO (Laser Interferometer Gravitational-Wave Observatory)project to the concept of a nonplanar ring oscillator single-frequency YAG laser, followed by an amplifier. The team selected that very stable laser system for the initial LIGO, and extended it to the new Advanced LIGO. At Stanford, working with Professor Dan DeBra, we designed an isolation system to stabilize Advanced LIGO against terrestrial noise. We knew we had done our job when the Louisiana LIGO site first turned on and the instrument came up, locked on, and stayed locked for seven hours. Advanced LIGO was undertaking preliminary runs when graduate students left after midnight, but fortunately left the detector in data-taking mode overnight. An hour later, a signal 23 times the noise level swept through. Initially, most of us thought it was a test signal, so we didn't get excited. The LIGO team checked very carefully, and after two months they were sure it was real. For the first time, we heard a "whoop sound" as two black holes merged, starting at 30 Hz and chirping up to 240 Hz in 0.2 s. What boggles my mind is that the merger converted three solar masses of matter into energy that radiated as gravitational waves. 1.3 billion years later, the signal arrived at earth and was detected by LIGO as a disturbance in space time with an amplitude of one part in 10-22—about 1/1000 the diameter of a proton at 4 km (see figure). This was the first—but not the only—signal being evaluated by LIGO after three months of observation.
OSA: Where do we go from here?
RB: We can improve Advanced LIGO's sensitivity by about a factor of three, which will increase detection rate by 33, a factor of 27. With that signal rate, we can do science on mergers of black holes, and of neutron stars. We want to build an instrument on the other side of the Earth, in India, to better triangulate the direction of gravitational wave sources on the sky. The original idea of a large 5 million km space-based system called LISA proved too expensive, so we're planning a smaller geocentric LISA (gLISA), with three spacecraft a million kilometers apart that would cost on the order of $1 billion. It would look for frequencies below 1 Hz to see the gravitational waves from mergers of giant black holes with a million solar masses, not the tens of solar masses we just detected.
OSA: What other big international projects are coming?
RB: Thirty meter-class telescopes on the ground are in the early planning stages. They will gather 10 times more light than the 10 m Keck telescopes, and if everything goes well they will be completed in the 2020s. Today, the spectra of exoplanet atmospheres backlit by their stars are at the signal-to-noise margin, but a 30 m telescope will show much more detail, so we could analyze gases in exoplanet atmospheres.
Laser-based yellow guide stars and adaptive optics have improved resolution of the Keck telescopes by more than an order of magnitude. But space-based telescopes get you above atmospheric absorption. That's the goal of the 6.5 m infrared Webb Space Telescope, to be launched in 2018. My dream is a phased-array optical telescope in space with an effective diameter of perhaps a thousand kilometers. By optically connecting a set of antennas and phasing them very carefully, we can achieve super-high resolution outside the atmosphere to study the spectra of exoplanet atmospheres. To do this, you need to build an array of drag-free satellites. At Stanford, we're already working on an array of three drag-free satellites for the gLISA mission.
Another surprising laser application now being evaluated is launching satellites into orbit. Average laser power of 10 to 20 MW should provide enough thrust to put a cubic-meter satellite into low-earth orbit. We're rapidly approaching megawatt average power in commercial products. Diode-pumped fiber and solid-state lasers have wall-plug efficiencies near 50%. Imagine replacing all the chemicals in rocket fuel with an electrically powered laser. That's a much cleaner, easier way to put matter in orbit. That's in the future, but probably this century.
OSA: What about other big optical research instruments?
RB: Stanford turned on a coherent x-ray laser in April 2009 that is now being replicated in Japan and Germany. These accelerator-driven free-electron lasers emit in the 1 Å or 10 keV range. They're big machines—the Stanford one takes a kilometer of accelerator and 100 m of undulator. At first they were like the early lasers, which Art Schawlow called "a solution looking for a problem." But now, coherent x-ray lasers are doing amazing science in the hard x-ray range and their time is oversubscribed.
Our lab, in collaboration with SLAC, is conducting research on a future particle accelerator driven by a laser, not by microwaves. A laser accelerator could drive an undulator to make x-rays, and all of this would take place on a tabletop. Two years ago, we were very fortunate to report real progress on laser-driven accelerators in Nature. That led to support from the Gordon and Betty Moore Foundation for a collaborative international research program on Dielectric Laser Accelerators (DLA). The goal of that five-year program is to build a fully operational accelerator, and then hand everything we have learned to accelerator labs in Europe and the U.S. We have a team of 20 people working on the accelerator science, inventing things, putting together a structure that efficiently couples the laser light to the electrons, and accelerating electrons at near 1000 MeV/m gradient for the first time. We use a dielectric, silicon or silicon dioxide, as an accelerator material and drive the accelerator structure with an off-the-shelf mode-locked 100 fs laser. It's been a lot of fun, and it's got a lot of room for creativity and discovery. In the end, this tabletop accelerator will really change how we get access to x-ray radiation and how we apply accelerators to science and to medicine.
ROBERT L. BYER, a professor of applied physics at Stanford University, has published more than 400 papers, and holds over 50 patents in lasers and nonlinear optics. He was President of The Optical Society in 1994, and is a fellow of OSA, IEEE, the American Physical Society, and the Laser Institute of America. He received OSA's R. W. Wood Prize in 1998, and was honored with the Ives Medal and prize from the OSA in 2009.
The Optical Society celebrates a century of innovation
Throughout a century of breakthroughs, The Optical Society has brought together the best minds in optics and photonics to light the future. This series reflects on that history and looks to what innovations lie ahead. For more information, please visit http://osa.org/100.