Photonic Frontiers: Microscopy: New twists on superlenses improve subwavelength microscopy
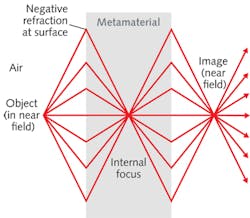
Subwavelength microscopy has come a long way from its early days as an exotic concept. The diffraction limit still rules for conventional bulk optics, but resolution can be pushed below half a wavelength in a couple of ways. Metamaterials and special instruments can achieve true super-resolution by directly recording transmitted, reflected, or emitted light. Alternatively, special processes using fluorescence, nonlinearities, or other techniques can achieve functional super-resolution.1 The latter "is more like signal processing. You acquire an image and try to improve [it]," says George Eleftheriades of the University of Toronto (ON Canada).
So far, the most practical techniques are functional ones, such as stimulated emission depletion (STED) microscopy, developed by Stefan Hell of the Max Planck Institute for Biophysical Chemistry (Göttingen, Germany).2 STED allows subwavelength resolution of a fluorescent spot by de-exciting fluorophores surrounding it. Its major applications, like those of other functional superresolution imaging, are in biomedicine. For example, in June 2014, PicoQuant (Berlin, Germany) added STED to its time-resolved MicroTime 200 confocal microscope, improving resolution to well below 100 nm.
Scanning near-field optical microscopy (SNOM) offers true super-resolution by moving a tiny aperture across the near field, but is too time consuming for many applications. Metamaterial devices, such as the superlens devised by Sir John Pendry of Imperial College (London, England), can observe much larger areas at one time.3 Thanks to that advantage, and the flexibility of true super-resolution, superlenses are coming on fast, although they still face major challenges, including limited bandwidth and resolution depth and special material requirements.
Superlenses and evanescent waves
The superlens concept is based on metamaterials in which both electric permittivity and magnetic permeability are negative at certain wavelengths. That gives them a negative refractive index, so they can capture evanescent waves that contain information needed to produce subwavelength images, but are not captured by conventional optics. As shown in Fig. 1, a superlens bends light entering it backward, forming an image plane inside it and another on the opposite side.
The most familiar evanescent waves are those that leak through a surface where total internal reflection occurs. They can be detected very close to the surface, but decay exponentially with distance from the surface, so they can't carry energy away. Pendry's superlens can achieve subwavelength resolution by focusing the waves near the surface. Negative-index materials "can actually amplify evanescent waves and thus restore high-resolution details which are inaccessible by classical imaging systems," writes Eleftheriades.4
A slab of negative-index metamaterial can serve as a superlens, which essentially produces a time-reversed wave with a negative frequency relative to the original, creating a super-resolution image, says Stephane Larouche of Duke University (Durham, NC). Negative refraction also reverses Snell's law and focuses rather than disperses evanescent waves. The metamaterial has subwavelength resolution over a large area, unlike SNOM, which can address only one point at a time.
Superlenses have their drawbacks. Attenuation tends to be high, particularly for metal films that have negative permittivity at visible wavelengths. Metamaterial properties depend on resonances, so the negative index crucial for super-resolution imaging only exists across a limited band of wavelengths. Superlenses also are limited to the near field and cannot focus in three dimensions.
Broadband subwavelength imaging
In 2012, Thomas Taubner of RWTH Aachen University (Aachen, Germany) and his colleagues proposed making a tunable broadband superlens from graphene. Graphene layers can both support plasmons and guide them, making them suitable for use in metamaterials. Varying gate voltage, electric field, or chemical doping applied to the graphene changes its conductivity, allowing continuous tuning of graphene properties at infrared and terahertz frequencies.5
Imaging in graphene is weaker than true superlensing because it is not strongly resonant, but Taubner's study showed that graphene sheets would enhance evanescent waves for subwavelength imaging. The RWTH team predicted that two graphene layers could achieve resolution of about one-seventh wave (λ/7), and multiple layers could reach about λ/10. Most important, the team predicted that a graphene lens could focus to below the diffraction limit from the mid-infrared to the terahertz band. Tauber and his colleagues also say their concept could be generalized to a two-dimensional "conducting sheet lens" of semiconductor heterostructures. Results of experiments with their graphene multifrequency superlenses have yet to be published.
Hyperlenses and metalenses
Evanescent waves can be focused into the far field with a hyperlens, based on an anisotropic layered metamaterial. The simplest type is a stack of alternating metal/dielectric layers much thinner than the optical wavelength. The original proposal called for hyperbolic dispersion in the metamaterial, but eccentric elliptical dispersion is another alternative, write Dylan Lu and Zhaowei Liu of the University of California at San Diego (La Jolla, CA) in a review paper.6
Far-field imaging was a surprise to many, but several types have been demonstrated. Figure 2 shows how a hyperlens with hyperbolic dispersion can focus evanescent waves radially, effectively converting them into propagating waves that carry subwavelength information in the evanescent waves into the far field. The flat layers are bent so light travels radially through them and magnifies an object placed at the inner radial surface so it can be viewed on the outer surface with subwavelength resolution. The ratio of the radii at the inner and outer curved layers gives the magnification at the outer surface.A hyperlens cannot do such standard optical tasks as focusing plane waves or performing Fourier transforms. That requires a metalens, a dense array of identical resonators fabricated in the plane of a metamaterial to provide the phase compensation missing in a hyperlens. Metalenses built from such elements as "meta-atoms," plasmonic metal-insulator-metal waveguide couplers, or graded index patterns can provide "an exceptional combination of super-resolution and desirable functions of conventional lenses," write Lu and Liu.6
Many metalens designs are under study. Liu proposed using arrays of bidirectional planar plasmonic waveguide couplers, as shown in Figure 3, but has yet to demonstrate it. Nikolay Zheludev at the University of Southampton (England) used meta-atoms that form a metalens to focus 800 nm light onto an array of subwavelength 160 nm hot spots beyond the near field of the lens.7 Interference among light waves propagating through the meta-atoms caused an effect called "superoscillation" to generate the spots.Vladimir Shalaev's group at Purdue University (West Lafayette, IN) developed a different planar nanostructure; a 4 μm lens with concentric rings of nanoholes machined in a 30 nm gold film. The lens focused 676 nm light only a spot 2.5 μm away; 476 nm light was focused at 7 to 10 μm from the lens. Their proposed design can be machined onto the end of an optical fiber.8
Camille Jouvaud and colleagues at the Langevin Institute (Paris, France) made magnetic metalenses from arrays of split-ring oscillators with different resonant frequencies. Microwave experiments produced localized modes instead of extended ones, and they predict their approach would allow subwavelength imaging in the visible and infrared.9
New directions for subwavelength microscopy
Super-resolution imaging is still young, and new ideas continue to emerge. One that particularly intrigues Larouche is assembling optical metamaterials by a self-organizing bottom-up process rather than using the top-down approach of photolithography. His lab recently made a near-infrared metamaterial with 30 nm elements. "We used electron-beam lithography, which is a great approach for research," he said, but not for mass production. "That particular device took weeks to fabricate."
He was intrigued by a recent proposal by Zsolt Szabo of the Budapest University of Technology (Hungary) and colleagues to embed silver nanospheres in silica to make a composite metamaterial for subwavelength imaging. They calculated that with self-organizing unit cells smaller than 20 nm, a single- or multi-layer metal-dielectric composite metamaterial could achieve 100 nm resolution.10
Subwavelength imaging is still young, and development is so diverse that there is no room to cover it all, particularly the many variations on functional super-resolution. Big challenges remain, but real progress is being made in seeing the previously unseeable.
References
1. A. Neice, "Methods and limitations of subwavelength imaging," in Advances in Imaging and Electron Physics, pp. 117–140 (2010).
2. S. Hell and J. Wichmann, "Breaking the diffraction resolution limit by stimulated emission: Stimulated-emission-depletion fluorescence microscopy," Opt. Lett. 19, 780 (1994); doi:10.1364/OL.19.000780.
3. J. Pendry, "Negative refraction makes a perfect lens," Phys. Rev. Lett. 85, 3966 (Oct. 2000).
4. A.M.H. Wong and G. V. Eleftheriades, "Advances in imaging beyond the diffraction limit," IEEE Photon. J. 4, 586 (Apr. 2012).
5. P. Li and T. Taubner, "Broadband subwavelength imaging using a tunable graphene lens," ACS Nano 6, 11 10107 (2012); doi: 10.1021/nn303845a.
6. D. Lu and Z. Liu, "Hyperlenses and metalenses for far-field super-resolution imaging," Nat. Commun. 3, 1205 (2012); doi: 10.1038/ncomms2176.
7. T. Ray, E.T.F. Rogers, and N. I. Zheludev, "Sub-wavelength focusing meta-lens," Opt. Express 21, 7577 (Mar. 2013).
8. X. Ni, S. Ishii, A. V. Kildishev, and V. M. Shalaev, "Ultra-thin planar Babinet-inverted plasmonic nanolenses," Light: Sci. Appl. 2, e72 (2013); doi: 10.1038/lsa.2013.28.
9. C. Jourvad, A. Ourir, and J. de Rosny, "Far-field imaging with a multi-frequency metalens," Appl. Phys. Lett. 104, 243507 (2014); doi: 10.1063/1.4882277.
10. Z. Szabo, Y. Kiasat, and E. P. Li., "Subwavelength imaging with composite metamaterials," J. Opt. Soc. Am. B 31, 1298 (June 2014); doi:10.1364/JOSAB.31.001298.
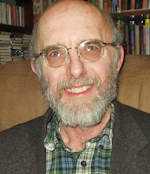
Jeff Hecht | Contributing Editor
Jeff Hecht is a regular contributing editor to Laser Focus World and has been covering the laser industry for 35 years. A prolific book author, Jeff's published works include “Understanding Fiber Optics,” “Understanding Lasers,” “The Laser Guidebook,” and “Beam Weapons: The Next Arms Race.” He also has written books on the histories of lasers and fiber optics, including “City of Light: The Story of Fiber Optics,” and “Beam: The Race to Make the Laser.” Find out more at jeffhecht.com.