Microscopy/Optogenetics: Boosting conventional microscopes for optogenetics
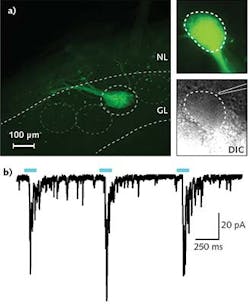
Optogenetics is a technique that uses light to control cellular activity within living tissue. This can range from wide-field illumination of brain tissue to stimulate a specific activity in a lab animal, to monitoring pathways at the single-neuron level. A key requirement for optogenetics is the ability to precisely direct light at the subject, often under a microscope. This article describes a modular system for accomplishing this task with popular microscopes from leading suppliers, and reviews how it has been successfully employed by neuroscience researchers at the University of Pennsylvania.
Foundational principles
Many biological processes are based on the use of ion concentration gradients between two hydrophilic regions separated by a lipid membrane. For example, energy in mitochondria is created in the form of a proton (pH) gradient, muscle cell contraction is mediated by sodium (Na+) and calcium (Ca2+) ion gradients, and nerve impulse transmission involves the flow of Na+ and potassium (K+) ions. Researchers have studied and manipulated these potentials for decades using tiny electro-mechanical probes in so-called "patch clamp" studies; typically, a micropipette electrode is inserted into a cell or forms a seal against the outer membrane. However, these electro-physiological studies have been limited in terms of spatial resolution and the number of sampling/manipulation points (usually to just one or two).
In recent decades, the development of fluorescent probes that are sensitive to local ion conditions (e.g., calcium ion concentration or pH) has enabled membrane potentials and gradients to be monitored in a non-contact, photonic manner, with a corresponding increase in sensitivity, speed, and spatial resolution. And now, the field of optogenetics has brought a photonic solution to the other side of the puzzle, namely manipulating these gradients.
The first optogenetics experiments utilized naturally occurring, light-activated proteins called opsins, which act as pumps for specific ions. Once the experimental principle was successfully demonstrated, research soon expanded with a range of channelrhodopsins and other classes of genetically encoded tools (e.g., C1V1). These optogenetic activators provide scientists a mechanism to turn target cells (e.g., muscle cells, cerebral cortex neurons) on or off in milliseconds, using light of the appropriate wavelength and intensity.
Controlled secondary illumination
Optogenetics has incredibly broad utility in life sciences research, from observing the activity of isolated cells, to stimulating specific actions in lab animals, to studying neural pathways in the cortex. For macroscopic applications, illumination requirements are often somewhat rudimentary. At the other end of the size spectrum, multiphoton excitation microscopes enable three-dimensional, in vivo studies at single-neuron resolution. Between these extremes, many experiments can be optimally conducted under a conventional, optical microscope, providing there is a means for accurately directing a secondary light source (laser, LED, or filtered lamp) at one or more targets in the field of view.
To support such work, a new generation of optomechanical modules provides a cost-effective method of integrating an externally controllable, secondary light source into conventional trinocular microscopes from leading manufacturers such as Zeiss, Nikon, and Olympus. The principle of operation is simple (see Fig. 1): A 45° beamsplitter is placed in the infinity space in the microscope body (tube). Specifically, a dichroic, bandpass, or other beamsplitter is held in a compact slider assembly that mechanically dovetails between the microscope body and the trinocular head. Once incorporated in the microscope, the slider mechanism allows the beamsplitter to be inserted and retracted from the microscope optical path, or even swapped for another beamsplitter, without any mechanical disruption or disassembly required.The compact collimator is configured with a standard FC-female input socket, and can thus accept any light source delivered via a single-mode fiber. A double-flexure tip/tilt mounting arrangement provides simple adjustment of the focused spot position—with minimized backlash, compared to alternative approaches, such as those based on dual-axis translation. The sensitivity of the spot adjustment in the focal plane again depends on the power of the objective, but is independent of the microscope tube length. When using a 20X objective with the differential screws supplied as standard with this setup, the relationship is approximately 110 μm per screw revolution for the coarse adjustment and 9 μm per revolution for the fine adjustment. Additionally, the flexure mount accepts motorized actuators for those desiring automated control.
Another advantage of using an optical microscope module that itself consists of several sub-modules is cost-effective flexibility. For example, the beamsplitter slider module can be used on its own to create fixed, 45° bidirectional access to the microscope infinity space. This enables the use of an additional eyepiece, camera, or other imaging device; e.g., with a polarizing or bandpass filter.
Investigating neural circuit function
The University of Pennsylvania Mahoney Institute for Neurosciences, founded in 1953, fosters cross-campus and cross-disciplinary research, including cellular and molecular neuroscience; development, regeneration, and plasticity; systems neuroscience; behavior and cognition; the pathology of brain disease; and computational neuroscience. Professor Minghong Ma and Wenqin Luo of the University's Neuroscience Department use this new modular setup in some of their research. They explain, "The neural system is fascinatingly complex. Any given part contains multiple types of neurons and each type connects with different parts of the brain. In the past, electrical stimulation has been used to evoke membrane potential changes in related neurons. But optogenetics allows us to take this to a much higher level of detail and understanding. Using different promoter genes, light-activated ion channels such as channelrhodopsin 2 (ChR2) can be selectively expressed in just one particular cell type and their functional connectivity with other cells can be teased out."
Ma and Luo's research groups are using a combination of optogenetic stimulation and patch clamp sensing to investigate, in ex vivo tissue, how the different cell types are synaptically linked in the olfactory system and the spinal cord, respectively. They stimulate ChR2-expressing neurons with 470 nm laser light and monitor resultant activity in other 'recorded' cells using whole-cell patch clamp methods.
These studies are performed under an Olympus upright microscope (model BX61) equipped with the IS-OGP optogenetics positioner. Professor Ma explains, "This workhorse multimodal microscope is nearly a decade old and is an important shared resource for several research projects. However, one limitation for optogenetics is that it has only one secondary light source input—a backside port configured for fluorescent illumination. Our work needs to leave this port dedicated for epifluorescence purposes. The IS-OGP provides a perfect method for introducing an additional laser for optogenetics, without disturbing or compromising any of the other microscope functions and capabilities."
In a typical experiment in Professor Ma's research, a 300- to 500-μm-thick tissue slice is put in the recording chamber and kept vital using a standard oxygenated solution. Epifluorescence is used to find ChR2-expressing neuron axons; differential interference contrast (DIC) imaging is then used to locate a suitable cell adjacent to the ChR2 axons. A patch clamp electrode is subsequently engaged with this cell so that changes in membrane potential can be observed, resulting from selective stimulation of the ChR2 axons using a few milliwatts of 470 nm laser light introduced to the field of view using the IS-OGP module. Typical data is shown in the Frontis.
Benefits for other microscopy experiments
When a new, fundamental methodology such as optogenetics is developed, it can impact many areas of research and often requires costly new tools. But sometimes, a component or sub-system can be implemented that upgrades existing and widely available instruments, such as the venerable upright microscope. These can enable cutting-edge research to take full advantage of the new methodology, without incurring substantial expense.
In addition to optogenetics, several other types of microscopy experiments also now require the ability to direct a spot of light at one or more locations in the field of view. Some of these require sophisticated illumination patterns-sometimes referred to as active illumination-generated by a spatial light modulator (SLM), often in conjunction with confocal imaging. But a simple method of directing an additional focused light source can often enable these to be conducted using a conventional wide-field microscope. Examples include photobleaching, uncaging, and selective gene ablation, to name just three.
Photobleaching refers to the loss of fluorescent properties in a probe or dye following intense and/or prolonged illumination. Although traditionally an unwanted complication in many experiments, sophisticated (i.e., spatially selective) photobleaching is now an important niche tool for cell biologists and others. Uncaging refers to compounds that release specific ions when triggered with light of an appropriate wavelength. For example, calcium ions can be locally generated for a host of applications by irradiating the compound NP-EGTA with ultraviolet light near 350 nm. And in studies of gene expression pathways, selective gene ablation involves using a focused spot of light from an ultrafast laser amplifier to irreparably damage one or more in simple organisms.
John Wingerd | Senior Mechanical Engineer, Siskiyou Corporation
John Wingerd is senior mechanical engineer with Siskiyou Corporation (Grants Pass, OR).