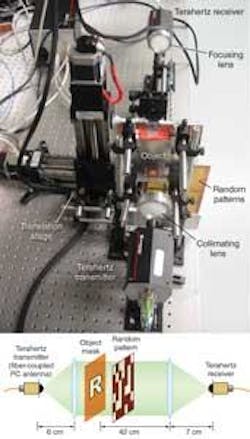
WAI LAM CHAN, DHARMPAL TAKHAR, KRITI CHARAN, KEVIN F. KELLY, RICHARD G. BARANIUK, AND DANIEL MITTLEMAN
A new approach to terahertz imaging, based on the concept of a single-pixel camera, promises an improved terahertz camera that will overcome disadvantages of current imaging systems, while providing superior speed and high detection ability.
Located between the microwave and near-infrared regions of the electromagnetic spectrum, terahertz radiation has many attractive properties for imaging applications.1 Similar to radar (microwave), terahertz systems can use either a broadband pulse or a narrowband signal for imaging. But, unlike with microwaves, terahertz image resolution can be submillimeter in dimension. Terahertz images can provide the ability to “see through” many types of plastics and clothing to find metallic objects, and unlike x-rays, the radiation is benign. Thus, security-related applications are one of the major focus areas in the terahertz community. Also, the high sensitivity of terahertz signals to the water content of materials provides important information for a variety of applications in food inspection, biological imaging, and more. The hyperspectral information from terahertz spectroscopy can be used to determine the chemical composition of many polycrystalline solids; applications include investigation of pharmaceutical materials and plastic explosives.
Existing approaches
Because of the wide range of possible applications, terahertz imaging systems are the subject of intensive research. Yet, each of the existing implementations has its own disadvantageslow speed, lack of sensitivity, large size, complexity, and so on. One traditional method involves a raster scan of the object to be imaged in front of the terahertz beam, while measuring and recording the transmitted (or reflected) terahertz wave at each scan position. This mechanical scanning is slow; the acquisition time scales with resolution and the scan area. State-of-the-art technology takes around six minutes to scan a 100 × 100 mm area at a 0.25 mm resolution.2
Other approaches, such as that pioneered by researchers at the Massachusetts Institute of Technology (Cambridge, MA), involve the use of focal-plane techniques, similar to the pixel array inside a digital camera, to achieve real-time terahertz imaging.3 However, these systems tend to have higher complexity and operational cost. For example, available array detectors, such as microbolometer arrays, are relatively insensitive to terahertz radiation, so a bright source is needed. Single-shot electro-optic sensing using crossed polarizers and a CCD camera also allows video-rate terahertz imaging, but this method requires a large and costly amplified femtosecond laser system.4 Clearly, novel imaging approaches are needed for improving the performance of terahertz imaging.
Single pixel sensing
For practical, time-critical applications, a terahertz imager should not require raster scanning of the object or the terahertz beam. In addition, one would like to preserve the superior detection sensitivity of single-point detectors such as photoconductive antennas (rather than the lower sensitivity provided by existing multipixel arrays). Based on the idea of a single-pixel camera, our approach offers a route to achieve both of these objectives. The rationale behind the high acquisition speed of the single-pixel terahertz camera is twofold. First, this camera performs compression simultaneously with image sampling by modulating the terahertz beam with a set of random patterns, a technique enabled by the theory of compressed sensing. This imaging scheme requires significantly fewer samples than the total number of image pixels to fully reconstruct an image, thus speeding up the acquisition process. Second, the speed of current terahertz time-domain systems is restricted by raster scanning. Spatial modulation of a free-space terahertz beam, either optically or electronically driven, can be much faster.
The principle behind applying compressed sensing to the design of imaging systems is analogous to a two-step image encoding/decoding process: one can view image acquisition as the encoding step, and image reconstruction as the decoding step. For image acquisition, the goal is to efficiently capture the information about an object into a small set of measurements. For image reconstruction, compressed sensing relies on the common spatial structures in real-world images. Based on the knowledge of these spatial structures, the reconstruction scheme “decodes” the small set of measurements to recover a full image of the original object through an optimization procedure.
In general, when the number of measurements is significantly smaller than the total dimension of the image, the image reconstruction problem is undetermined; that is, it has more than one solution. The compressed-sensing encoding procedure (image acquisition) needs to ensure a one-to-one mapping between the class of images with certain spatial structure and the measurement space to allow exact image recovery. A particular choice of encoding is to use a weighted sum of all of the image pixels as each measurement, with weights of zero and 100% at random pixel locations.5 This binary filtering can be easily implemented optically by randomly blocking a subset of the pixels in the image and collecting the remaining light with a single lens.
Experimental results
A prototype single-pixel terahertz camera uses a set of fixed masks that are mechanically positioned between the object and the terahertz detector. The terahertz beam, after passing through the object, travels through a random subset of pixels on each mask to reach the detector (see Fig. 1). In this demonstration, the object is made of copper tape on a transparent plastic plate, with a hole shaped as the letter “R” (see Fig. 2). The masks each consist of a random pattern of opaque 1 mm2 pixels printed on standard printed-circuit boards, which are transparent to terahertz radiation. These are mounted onto a motorized translation stage, which ensures accurate alignment when changing from one random pattern to another.The detector collects one measurement for each mask, consisting of the superposition of the radiation transmitted through the random subset of transparent pixels on each mask. Using a number of measurements that is smaller than the total number of pixels in the image, the reconstruction algorithm obtains a 32 × 32-pixel image of the object with the correct dimensions and details. This reduction in the total number of required measurements is a significant advantage for terahertz imaging because, for a pixel-by-pixel acquisition scheme, this is the rate-limiting step for image formation.
Other advantages of this approach include hardware simplicity and versatility. With a continuous-wave terahertz source, such as a terahertz quantum-cascade laser, this camera can use a sensitive single-pixel detector, such as a Golay cell, instead of a detector array, thus reducing the source power requirement. With a pulsed source, this camera can extend its imaging capability to capture spectral phase and other hyperspectral features. Preliminary experiments have demonstrated the estimation of the thickness of a plastic plate from the extracted phase delay acquired by the terahertz beam.
The next generation of this camera will replace the random-pattern masks with a terahertz spatial modulator, driven either by electronic or optical means. This should permit us to modulate the terahertz beam very rapidly, without the need for any mechanical moving parts. As a result, we anticipate that we will be able to acquire sufficient information for image reconstruction at a rate compatible with video imaging.
REFERENCES
1. W.L. Chan and D.M. Mittleman, Rep. Prog. Phys. 2007(70) 1325.
2. D. Zimdars, Proc. SPIE 2005 (5692) 255.
3. A.W.M. Lee et al., Applied Phys. Lett. 89, 141125 (2006).
4. Z. Jiang and X.C. Zhang, IEEE Trans. Microw. Theory Tech. 47, 2644 (1999).
5. M.F. Duarte et al., IEEE Signal Processing Mag. 25, 83 (2008).
WAI LAM CHAN and DHARMPAL TAKHAR are graduate students, KRITI CHARAN is an undergraduate student, KEVIN F. KELLY is an associate professor, and RICHARD G. BARANIUK and DANIEL MITTLEMAN are professors at the Department of Electrical and Computer Engineering, Rice University, Abercrombie Building-A204, 6100 Main St., Houston, TX 77005; e-mail: [email protected].