Monocrystalline cells dominate solar photovoltaic industry, but technology roadmap is far from certain
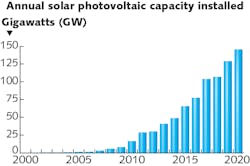
The solar photovoltaic (PV) industry continues to be a sector characterized by strong double-digit growth, with annual deployment levels in the 100–200 gigawatt (GW) range. Today, end-market demand is firmly a global affair and viable in the absence of government subsidies. Manufacturing of components across the upstream value chain—including ingots, wafers, cells, and modules—is dominated by Chinese companies, with a handful of Western companies striving to remain competitive.
However, this new phase of Chinese domination is no longer based upon scaling legacy Western-originated process flows within low-cost/low-overhead manufacturing facilities. It is now centered on mega-sized (10–20 GW) manufacturing facilities, a greater use of domestic tooling, and implementing frequent changes to increase efficiencies, throughputs, and cost-reduction efforts.
This article explains how these changes have resulted in solar-module power ratings moving from 250 to 260 W as mainstream in 2015 to levels from new variants today in excess of 500 W. Discussion is presented highlighting a sector that is now dominated by a small group of Chinese majors that can sustain 15–20% production gross margins almost independent of market conditions, with each seeking to impose different versions of a technology roadmap for the industry as a whole.
Understanding how the solar industry arrived at this current phase of manic technology change implementation is explained, with accompanying narrative on what may unfold going forward.
Growth drivers in a 100 GW-plus annual deployment era
Annual deployment of solar capacity has been doubling every four years for the past couple of decades, reaching 125 GW in 2019. Initially a sector that had relatively strong contributions from rooftop installations (residential and commercial buildings), solar in 2020 is all about large-scale solar farms typically in the range of 100 MW to 1 GW, spread across large areas of the countryside. This ground-mount demand is utility sector-stimulated and often mandated by governments or utility providers as part of long-term environmental necessity.Solar can now be deployed faster and more cheaply than almost any other energy type, creating long-term demand drivers that are prime for external investment: shareholder returns on upstream manufacturers’ earnings, and long-term de-risked revenue streams based on selling energy to off-takers.
China, the U.S., India, and Japan continue to add significant levels of new capacity each year; in 2020 alone, China is likely to add more than 40 GW. Europe collectively is a 20 GW proposition, with countries in Southern Europe (in particular Spain and Portugal) now largely operating subsidy-free. Australia has now become a key focus, with new gigawatt-scale additions coming from large-scale utility projects, adding to the strong adoption of residential systems of the past few years. Some of the strongest growth, however, is coming from specific countries in Southeast Asia, Latin America, and Middle East countries.
The rise and fall of p-type, multicrystalline-based solar cells
When the solar industry grew from a 10 GW annual market to 50 GW between 2010 and 2014, the mainstream technology was based upon the use of multicrystalline silicon (mc-Si) wafers, sliced from p-type casted silicon ingots (bricks) into 6 in. (156 mm) square solar cells. Until 2016, modules assembled using these solar cells accounted for about 70–75% of annual deployed solar capacity.During this period, p-type mc-Si solar cell efficiencies were typically in the 17.5–18% range. When packaged into modules (routinely using 60 cells), module powers were rated at 255–260 W. Utility solar sites and large commercial rooftop installations were the key markets targeted by this mainstream module type.
The remaining 25–30% of module production comprised higher-efficiency silicon-based solar cells (n- and p-type monocrystalline silicon) and thin-film variants that accounted for about 5% of global supply.
The benefits of manufacturing p-type mono solar cells were obvious even at this time; cell efficiencies of 19% to 19.5% translated to module powers of 270 to 280 W. Moreover, many of the advanced cell concepts—known within the research community dating back to the early 1980s—were dependent on higher-quality mono silicon wafer substrates being used.
However, there was limited desire to change to mono as the mainstream technology. This was due to the cost structures achieved from companies making mc-Si wafers being significantly lower than those pulling silicon ingots (rods) using the traditional Czochralski (CZ) method adopted from the semiconductor industry.Additionally, the difference in power output between p-type mono and p-type multi modules was rather minimal, at about 5–7%. This simply did not merit any wholesale change to mono at the time.
What happened next, during 2017 through 2019, completely changed the landscape of solar cell and module production.
The emergence of LONGi Solar, bifaciality, and 500 W panels
Since 2017, the solar industry has witnessed a deluge of technology changes across the whole upstream value-chain, from ingot pulling, wafer slicing, and cell production to final module assembly. Barely pausing for breath, this three- to four-year period has seen the manufacturing landscape change almost on a quarterly basis, with no one really knowing what, if any, end goal is being pursued.
To explain this best, it is necessary to look at the different driving forces in play during this period of change, and what has led to module powers being produced today with power ratings well above 500 W.
The key driver for change came from the emergence of a leading Chinese company (LONGi Solar) that completely changed the landscape for mono wafer production/supply by making mono ingot pulling a commoditized activity. LONGi Solar added 20 GW of mono ingot/wafer capacity in China between 2013 and 2018, operating with a cost structure that enabled matching multi wafer prices while reporting 20–30% gross margins. LONGi Solar would soon be joined by another Chinese company, Zhonghuan Semiconductor, that enacted similar mono ingot/wafer expansion plans rapidly. By 2017, cell makers could finally choose between mono and multi wafers without any excessive materials cost contribution.Around the same time that LONGi Solar was constructing its multigigawatt mono ingot/wafer factories in China, cell producers had started to make a key process-flow change that had been delayed in implementation for about 30 years—the use of transparent dielectric materials deposited on the rear surface to replace screen-printing of aluminum. This architecture had been designated the passivated emitter and rear contact (PERC) cell by researchers at the University of New South Wales (Australia) in the mid-1980s. Today, the acronym appears on almost every silicon-based solar module sold globally.
Implementing the PERC concept proved ideal for mono-based wafers, and very quickly mono cell efficiencies were averaging about 15% above multi cell types. However, making mono-based cells with the new PERC process flow also enabled additional changes, ultimately allowing the rear surface to be used also for light absorption (bifaciality).
The industry moved rapidly to both p-type mono wafer use and PERC cell manufacturing, through upgrading existing cell capacity and making mono the basis of all new capacity coming online in China and across Southeast Asia. This surge also became the first major use of laser tools in solar manufacturing, needed to create the contact openings through the passivation stack deposited on the rear surface. For the first few years, European-based laser integrators saw strong order books; however, by 2019, Chinese laser tool suppliers (in particular Wuhan DR Laser Technology) had captured over 90% of the market for lasers-in-PERC.
By 2018, p-mono-based PERC cells were making up about half of annual solar production. In 2020, this figure is likely to be closer to 75–80%.
However, moving to p-type mono wafers and applying the PERC process in cell production does not take module powers into the 500 W-plus levels many are reaching today. This final part of the technology-change jigsaw is something that has been rapidly thrust upon module users, mainly in the past 12–18 months.
The shift from 250 to 300 W panels to 500 W-plus is due to two factors—increasing the size of the wafers (cells) and using a greater number of these cells in an assembled module. It is perhaps not the most subtle technology roadmap node the solar industry has witnessed. However, it has created levels of uncertainty not seen before.
The reason for the confusion is that there appears to be no standard wafer dimensions or module sizes being adopted across the leading 10–20 module suppliers. A range of larger wafer formats is being pushed now—in particular, 166 mm and 210 mm. In turn, the number of cells per module is also variable, creating module size variations that result in utility-scale projects using modules anywhere between 400 W and 600 W, not to mention modules that are either mono-facial or bifacial light absorbing.
For years, the industry was hungry for manufacturing technology changes. Now, these are happening in droves and being driven in the absence of any industry unity. The net result is total confusion in what, if any, technology roadmap is being followed, and where the next change is coming from.
n-type cells still seeking to brush off niche label designation
The ultimate technology change that many in the industry have assumed for years will happen by default is the move from p-type wafers to n-type. The n-type substrates have superior properties that have been shown by early movers, such as SunPower and Sanyo (now Panasonic), to result in efficiencies well above the best-in-class p-type variants. Additionally, n-type modules suffer less than p-type modules when operating at elevated temperatures akin to the normal environments that solar operates within.
However, neither SunPower nor Panasonic were able to make the gigawatt to 10 GW-plus capacity expansion moves in the past 4–5 years that were essential to remain a top-10 global supplier. Indeed, SunPower has recently carved out its upstream operations into a newly listed entity, called Maxeon Solar, whose growth plans are based on using p-type cells made in China.
Most of the leading Chinese module suppliers today have established pilot-line or initial mass production of n-type cell types, mainly as a diversified play or defensive in nature in case any major breakthrough in production costs is achieved by a peer-group competitor. The two n-type options seeing strong investments today are heterojunction-based cell types (analogous to Sanyo’s original technology play) and a more simplified n-type class often referred to as passivated emitter rear totally diffused (PERT) or tunnel oxide passivated contacts (TOPCon). While a few companies have established capacities at the 2 GW level today using the PERT/TOPCon variant, it remains unclear if n-type will really move away from its perennial niche tag that has characterized its time in the industry to date.
Beyond n-type options (based on heterojunction or PERT/TOPCon), the topic of tandem or multijunction cells (even using perovskite structured compounds) is always somewhere in the mix. However, it would be fair to conclude that activity here is firmly in the research labs, but still important to keep tabs on over the next five to 10 years.
Restoring some form of roadmap alignment may not happen until 2022
Current manufacturing activities and capital expenditure from the top 20 module suppliers today suggests that 2020 and 2021 are going to result in a further 12–18 months of p-type mono-based module production growth and module format variance. In this respect, it is very hard to see any major changes in terms of n-type technology adoption for some time. Segments of the industry retain the hope that n-type adoption is a matter of when, not if. However, the more pragmatic voices are of the opinion that p-type is the sweet spot for solar and has another five years to run before any major process-flow changes are needed.
Perhaps the introduction of the more-esoteric cell types (tandem/perovskite) may stand a better chance of adoption if the pragmatic viewpoint comes to fruition. These more research-centric structures certainly need more time to get out of the research lab, and by the time the industry is hungry for n-type dominance, timing could be in their favor.
In the meantime, technology in solar is all about p-mono PERC and bragging rights about how much silicon can be assembled into a single module format, breaching power-level thresholds that were the basis of science fiction just a few years ago.
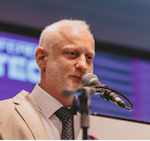
Finlay Colville
Dr. Finlay Colville has been Head of Research at PV-Tech and Solar Media Ltd since 2015. Before this, he spent 5 years as VP and head of solar at market research firm NPD Solarbuzz, and 10 years in various sales and marketing roles at Coherent, Inc. Dr. Colville holds a BSc in Physics from the University of Glasgow and a PhD in nonlinear photonics from the University of St. Andrews.