Future Photonics: 5G—Optics will be indispensable for 5G networks
The first products in the fifth generation of mobile phones made their debuts in 2019, but a full rollout of the technology will take many years. Current plans include providing connections for other technologies still in their early stages, notably the Internet of Things (IoT) and autonomous vehicles. 5G is a work in progress rather than a fully defined standard. It includes goals such as millisecond latency and 99.9999% reliability, which for now seem more aspirational than attainable. Nonetheless, 5G is shaping the future of communications.
Most descriptions of 5G are dominated by the words wireless and mobile. Yet optics will remain the data-transport backbone of the network, embedded in submarine and terrestrial cables, data centers, and transmission lines running to wireless transmitters and to buildings. 5G may even push fiber optics all the way to homes in Europe and North America, where only 15% are connected to fiber—although some 40% of homes are passed by fiber—far behind subscription rates of 80% in South Korea and 90% in Singapore.
Goals of 5G
The top three major goals for 5G usually are listed as:
Enhanced mobile broadband (EMBB): Providing peak data rates of 10 Gbit/s or more. This is often mentioned together with emerging technologies such as augmented or virtual reality or “ultra-high density” video, but it’s really about delivering all the bandwidth that customers want for all their information needs. This covers everything from old-fashioned audio telephony and text messages to telework connections, game consoles, wall-sized video screens with high dynamic range, and high definition, high-performance personal video and audio. This can be thought of as a souped-up Internet, perhaps branded as “mobile” but really providing fixed broadband as well.
Ultra-reliable low latency communications (URLLC): Services with reliability of 99.9999% and latency in the millisecond range. Both will be needed to track and control autonomous vehicles as well as everything else in their vicinity, including other cars, bicycles, pedestrians, construction sites, and even trees. Reliability and quick response also will be vital for other devices, such as home medical equipment.
Massive machine type communication (mMTC): Transporting data among the IoT, a large and varied family of devices expected to proliferate to densities of hundreds of thousands per square kilometer. Many homes already have connected thermostats, security cameras, and medical devices, but planners envision many more that will be chattering to each other incessantly without human intervention.
All this is in the future. Mobile-phone companies began selling 5G phones in 2019, but they will need a couple of years to install enough transmitters to give dramatically better bandwidth. Potential applications such as fully self-driving cars and virtual reality are running behind earlier plans. But even if those applications never get off the ground, more capacity will be needed to keep up with existing trends, like streaming video and higher screen resolution.
The mobile broadband spectrum
5G will expand its bandwidth with new technology and by expanding the part of the radio spectrum devoted to mobile communications. Different parts of the spectrum serve different needs. Frequencies below 1 GHz, once occupied by broadcast television and earlier mobile phones, offer wide-area coverage but limited bandwidth for slow data rates from the IoT. Frequencies of 1 to 6 GHz, used for satellite links, mobile phone, WiFi, and other purposes, offer more bandwidth, but their range decreases with increasing frequency, so a 2.5 GHz WiFi has more range than 5 GHz. The biggest source of new bandwidth is above 6 GHz, with the U.S. using 27.5–28.35 GHz, 37–40 GHz, and 64–71 GHz. Those higher frequencies have more bandwidth but their range is more limited, so the transmitters must be close to users. Gaming and high-resolution video are expected to be major uses.
Today’s cellular networks typically have cells 2 to 50 km across, with the largest in rural areas. Microcells 0.4 to 4 km are used in urban areas to serve higher population densities. Transmission losses are much higher in the 28 GHz band, so 5G will use microcells and smaller picocells tens of meters across and femtocells, about the same size but at lower power. That will bring antennas to within a few doors of homes in many areas.
All those cells require backhaul connections to the network, generally through a central office that connects signals from many cell towers. As of 2015, about 90% of cellular backhaul was through copper cable, 6% via fixed wireless microwave, and 4% through fiber. That mix is evolving, as 5G’s need for more bandwidth is making copper inadequate.
Figure 1 shows a simplified version of the emerging network. Small cells in the 28 GHz band will distribute broadband signals short distances, with large cells operating over larger areas at frequencies below 6 GHz. Wireless signals reaching antennas or base stations will be transmitted through fiber or other links to central offices, either in radio format or digitally. Equipment at the central office will decode radio signals and process others. Those requiring low latency will be routed to a local data center; others will be routed to other destinations at remote data centers or network core. Fiber will transport much of the data, but it will compete with fixed wireless microwave and free-space laser links. Those systems are now in development, as described below, with the choice depending on cost/performance benefits.
‘Pluggables’ to upgrade the network
While 5G hardware will not reach directly into the network core, it will bring a flood of data traffic that will require major expansion of transmission capacity. Growth in the fiber transmission system will be divided between datacenters and the external fiber network, says Bill Gartner, senior vice president/general manager of Cisco’s Optical Systems and Optics Group. Fiber is plentiful inside datacenters, and transmission distances are short: no more than 10 km, and often shorter. “Every router or switch port has its own dedicated fiber; if a new switch or router is added, additional fiber is added to terminate the new ports,” says Gartner. This will require increasing port densities and large-scale deployment of new high-speed optics.
Advances in silicon integrated circuits have steadily decreased the cost per bit for electronics, but the cost per bit of optics has not decreased as rapidly, says Gartner. Thus, as the bit rate increases, the cost of the optics becomes a larger portion of the hardware cost. Optics account for 10% of the hardware cost in a 10 Gbit/s datacenter network, but he says that at current rates, the optics will exceed half the hardware cost when data rates reach 400 Gbit.
Cisco’s approach to control optics costs is to push development of “pluggable” optics, which are standardized modules packaging optics and electronics to convert signals between optical and electronic form (see Fig. 2). One end of the module is an electronic module that plugs into the electrical interface on the router, while the other is an optical connector connecting to the output fiber. Modules generate optical signals in formats that optimize performance over specific distances, such as 500 m or 2 km. Datacenter transmission is limited to about 10 km, so the pluggables use direct detection of ones and zeros rather than coherent transmission to limit costs.In the long-haul transmission network outside datacenters, fiber is scarce, data rates are very high, and repeater spacings are more than 80 km. In those systems, the preferred signal format combines dense wavelength-division multiplexing (DWDM) and coherent transmission to increase the capacity of installed cables. Gartner says that pluggable coherent optics do not bulk up the connections at routers or switches, and allow carriers to automate network operation.
Radio over fiber
At some point on the way from the network core to the antenna, digital data must be converted into radio signals for wireless transmission. This can done by installing the radio-generation equipment at the base station, which it can convert output signals from data into radio and the analog signals received from the antenna from radio into data. However, that requires installing the equipment at radio transmitters, which can be expensive and can add to maintenance costs.
An alternative, Radio over Fiber, converts the data into radio signals at a central office or other regional facility and converts the radio signals into a form that can be transmitted optically through a fiber to the base station. A centralized software-defined network (SDN) can create a Cloud-Radio Access network to transport the signals to and from central offices and transmission stations. In practice, the biggest advantage of radio over fiber comes when antennas are receiving multiple radio signals that require sensitive equipment to process. The radio signals can be transmitted at the received frequencies, or converted to a lower intermediate frequency that is easier to transmit and can be processed further at a central office.
How far should fiber go?
One big question that remains unanswered is the best way to deliver signals to the closest wireless broadband transmitters. To deliver signals reliably in the 28 GHz band, antennas probably will need to be within 100 m, and concrete or brick walls can block such high frequencies.
Would it be better to bring the signals inside the house, in the form of fiber to the home (or premises for apartment dwellers)? Raf Meersman, CEO of consulting firm Comsof (Ghent, Belgium), thinks the best approach would be to combine building out 5G fiber backhaul with installing fiber to the home. At the FTTH Conference (Amsterdam, Netherlands; Mar 12-14, 2019), he said that 5G and FTTH are synergistic because of the density of high-frequency antennas needed. “Between 65% and 96% of fiber costs for 5G [backhaul] can be eliminated by rolling out an optimized and future-proof converged fiber network,” he noted.
Meersman bases his argument on models of urban, suburban, and rural areas that include various density levels for wireless cells. He found that total costs could be slashed by building a single network to deliver both FTTH and 5G 28 GHz broadband wireless services. “The extra investment needed to immediately make an FTTH network ready for 5G (even for a high density of cells) is only 1% to 7%,” he claimed.
It’s an interesting argument because, although only about 15% of U.S. households subscribe to fiber broadband, fibers pass about 40% of homes. If a carrier could add fiber drops to 5G antennas to an existing FTTH network, it could save a bundle in installation costs. Conversely, if the carrier had to install fiber close to homes anyway to install 5G antennas, it could recover some of the cost by adding FTTH connections.
However, fixed wireless links and free-space optics offer alternatives that could slash installation costs, at least in some areas. Fixed wireless has been used for previous generations of wireless base stations, and free-space optics had found some applications. However, both require line-of-sight operation and can be attenuated by weather.
Fixed wireless transmission in the radio and optical bands has been used for a long time, and offers a way to avoid high construction costs for transmission, but has suffered serious limitations from poor performance, licensing requirements, and high costs. 5G has opened up new possibilities in the radio band, including multiple-input, multiple-output (MIMO) transmission that gives more concentrated beams and high-frequency transmission, although the latter is limited by distance. A recent analysis by Suman Malik and Prasant Kumar Sahu at the Indian Institute of Technology (Bhubaneswar, India) found that free-space optics transmission also was vulnerable to group-velocity dispersion and chirp at long distance. They suggest that combining radio and optical free-space links could improve reliability, but would add to costs. Although wireless links could cut costs, they also face serious performance challenges.
A new alternative technology that uses free-space laser communication is putting constellations of small satellites into low earth orbit (for example, Starlink by SpaceX). In this scheme, ground stations will transmit signals up to the satellites, which will relay them around the world using intersatellite laser links to a point where they can be transmitted down to their destination. The goal is to bring broadband Internet to regions poorly served by terrestrial and submarine fiber cables.
Low latency and other challenges
The quest for millisecond latency poses another major challenge because it is a serious goal for applications from autonomous cars to home medical monitoring systems. The speed of light in glass is about 200,000 km/s, putting a hard minimum on the latency of a cloud computing center. Just the round trip to a data center a thousand kilometers away takes 5 ms, and that doesn’t count the processing time at either end. That time counts when steering a car at freeway speeds or in reacting to a possible medical event.
Reducing that latency will require building a mesh of datacenters across the country. They would not have to be big, but they would have to be fast. Researchers are looking at ways to ramp up the speed. In one experiment, Hwan Seok Chung and colleagues of the Electronics and Telecommunications Research Institute (Daejeon, South Korea) bonded together a pair of WDM channels at different wavelengths to transmit 25 Gbit/s packets in parallel with latency below 400 µs over 20 km to deliver data at 50 Gbit/s.
More challenges can be expected to emerge as 5G matures. The technology itself is sure to pose challenges, but the bigger challenge may be finding money for investments that will take years to pay off.
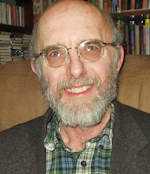
Jeff Hecht | Contributing Editor
Jeff Hecht is a regular contributing editor to Laser Focus World and has been covering the laser industry for 35 years. A prolific book author, Jeff's published works include “Understanding Fiber Optics,” “Understanding Lasers,” “The Laser Guidebook,” and “Beam Weapons: The Next Arms Race.” He also has written books on the histories of lasers and fiber optics, including “City of Light: The Story of Fiber Optics,” and “Beam: The Race to Make the Laser.” Find out more at jeffhecht.com.