Wireless communications is a restless field. Although the global 5G network is still taking shape, developers are already planning how to deliver more bandwidth and improve latency in the next-generation 6G network.
Planners say 6G will be the next big thing, noting it will deliver new wireless services including data links to a multitude of “Things” and augmented reality to people at holographic high resolution.
Generations of wireless networks
Mobile telecommunications have gone through generations of standards, each marking an important step in technology. The first widely used mobile phones were cellular analog systems, now called 1G. The digital 2G cellular networks, launched in 1991, initially offered texting followed later by low-resolution images and multimedia. The 3rd Generation Partnership Program (3GPP) was formed in 1998 to develop standards for 3G cellular mobile phones, designed to carry data for the fast-growing Internet. 3G opened the door for smartphones, starting with the Blackberry, designed for business users. The industry has since introduced a new generation every decade. 4G brought smartphones for everyone, 5G emerging around 2020 with even more bandwidth, with 6G targeted for 2030.
Gerhard Fettweis, Vodaphone chair professor of mobile communication systems at the Technical University of Dresden (Dresden, Germany), says the industry now introduces two generations of each new technology—the first for business users and the next generation expanding the market to consumers, followed by a new generation of technology for business. 3G brought smartphones and the Internet to mobile business users, with 4G expanding them to mobile consumers. He says 5G networks will provide a “tactile Internet” that gives professional users in fields from agriculture to medicine wireless real-time control of real and virtual objects in the Internet of Things.
Fettweis also notes that “6G must provide an infrastructure to enable remote controlled robotic solutions for everyone, the Personal Tactile Network. From 2030 on, with the launch of 6G, the old dream of mankind of robotic helpers easing our life will therefore become reality.” It’s a big, broad vision that seeks to realize concepts including augmented reality as well as personal robotic agents. Achieving that goal will require new technology that can deliver enormous transmission capacity, provide extensive interconnectability, and achieve nearly instantaneous latency.1
Importance of the physical layer
According to Michail Matthaiou, a professor at Queen’s University (Belfast, UK), the success of 5G can be attributed to the use of massive multiple-input multiple-output (MIMO) technology in the microwave band. Their main concern is the physical layer of mobile networks, the hardware that transports signals. Other layers of communication systems perform other functions, such as delivering voice, video, or data inputs into the system, converting those input signals into a suitable format for transmission, and multiplexing signals from various sources into a single data stream for transmission through the physical layer. Optics are part of the physical layer. Optical transmitters generate light signals that travel through fibers or the air for free-space communications. Radio and microwave signals from antennas travel wirelessly through the air. Electronic transmitters generate electrical currents that travel short distances through wires.
Fiber optics play important roles in the physical layer of 5G mobile networks (see Fig. 1). They provide high-capacity backbone transport for the global telecommunication system and between datacenters. They also provide backhaul between regional distribution nodes and the cellular towers that distribute wireless signals at radio frequencies. Passive optical networks can transmit radio signals in the 28 GHz band used by 5G networks to transmit from local antennas distributing high-frequency signals serving small “picocells.”
Challenges in scaling to 6G
Optics play a major role in scaling the physical layer of mobile networks to meet the goals of 6G networks. Matthaiou says this requires three major technological advances: transmitting signals at frequencies from the 28 GHz band used in 5G ranging up to the optical band, developing intelligent reconfigurable surfaces for antenna systems, and replacing conventional fixed cellular networks with cell-free networks based on massive multiple-input multiple-output (mMIMO) networks.
The three major bands envisioned for 6G are millimeter waves at 30 to 300 GHz, terahertz at 300 GHz to 3 THz, and the optical band, which includes much of the infrared (IR), all of the visible, and the long-wave end of the ultraviolet. Atmospheric absorption is a well-known problem for most of the millimeter-wave band, with most air attenuation ranging from 2 to 10,000 dB/km at 300 GHz to 10 THz.2 At least in the near term, that high attenuation limits transmission to short distances in most of the millimeter and terahertz bands. Obstructions such as foliage or walls can also block transmission.
The goal of intelligent reconfigurable surfaces is to revolutionize the structure of the antennas transmitting and receiving wireless signals. Antenna shapes determine how they radiate or collect radio waves, like the shapes of mirrors focus light. Phased arrays of simple antennas can focus incoming or outgoing radiation or light. A step beyond phased arrays are metamaterials or metasurfaces, comprised of subwavelength elements that combine to shape radiation in more complex ways. Intelligent reconfigurable surfaces add the capability of dynamically controlling the shape of the surface to change how it directs light or radiation, allowing the complex movable antenna to synthesize desired emission patterns and signals.
In radio communication systems, MIMO involves one array of multiple emitters transmitting signals to a second array of multiple collectors. Broadband fiber-optic communications use MIMO coding to transmit signals in high-order modes, but the number of possible configurations of the signal is small. mMIMO involves large numbers of input and access points, which serve as antennas.
Existing cellular communication networks suffer undesirable boundary effects near the borders between cells. The most familiar problems are weak signals at those boundaries and dropouts when moving between cells. mMIMO can create many access points distributed across a wide area, so signals could travel on multiple different paths, and weak signals could be added to give stronger combined signals. The more objects in the network, the more uniformly they can distribute signals over a wide area. Instead of the few large transmitting antennas in a current cellular network, each of the access points in a cell-free mMIMO would have multiple small, low-power antennas.
Optical wireless communications
Fiber-optic cables have long provided backbone transport and backhaul for wireless networks, but wireless optics were not used until 4G systems were deployed. However, the high frequencies of light waves make optical transmission more attractive for bandwidth-hungry 6G wireless applications.
Microwave frequencies limit the attainable bandwidth of wireless networks. The new WiFi6 wireless standard manages to achieve data rates to 9.6 Gbit/s by operating in parts of the microwave spectrum from 1 to 7.125 GHz. Optical wavelengths, with frequencies of hundreds of terahertz, promise much higher data rates for wireless applications.
The most mature technology is Li-Fi (light fidelity) proposed by Harald Haas, of the University of Strathclyde (Glasgow, UK), to cover wireless optical systems that use visible and IR light to provide two-way multiuser communications.3 Optical wireless generally works best with a line of sight between the transmitter and receiver, but it can work if light is spread and reflected diffusely. Non-line-of-sight operation is possible, but may require special technology and signal processing. Walls, doors, foliage, fog, precipitation, and other obstructions can block or strongly attenuate light, so in practice most wireless optical links cover only short to medium distances.4 Whether that limit is a bug or a feature depends on the application. Containing signals within a room or building can provide security in many situations, but could be a problem in a network serving multiple rooms or structures, or reaching people outside of the building.
LED sources are attractive for short and medium wireless communication because they can disperse light across wide angles to give desirable multipath diversity on short links, and their low power avoids the risk of eye damage. One early appeal of the Li-Fi system was the idea of modulating overhead LED light bulbs with signals that would spread through a room like radio waves. The optical illumination could provide the downlink from server to users, with individual user devices equipped with radio uplinks.
Kyocera SLD Laser (Goleta, CA) has demonstrated a new type of laser-based surface-mount device (SMD) Li-Fi source in which two gallium-nitride (GaN) diode lasers illuminate phosphors to generate white light (see Fig. 2). “The blue or violet laser sources illuminate the phosphor, which converts some energy to yellow for white light. The laser light undergoes Lambertian scattering, yielding a mixture of about 20% blue and 80% yellow” in the output of their LaserLight Li-Fi, says Paul Rudy of Kyocera.“The data rate is what's very important,” says Haas, who worked with Kyocera on the demonstration. “You can use it in daylight, with infrared and visible light. It works across different scenarios and can be combined with sensing.” That’s important because 6G will combine sensing, communications, and computing, he adds. “Maybe 20 years from now, wireless will be optical rather than radio.”
For now, Rudy says Li-Fi is attracting interest from users concerned that radio wireless signals could interfere with sensitive medical or other equipment or could leak secrets from secure facilities. The aviation industry also is interested because it worries that noise from radio bands the FCC recently opened to wireless communication could interfere with sensitive navigation equipment.
Latency and transport networks
Reducing network latency to submillisecond levels is high on 6G wish lists because it is too short for humans to perceive, which is crucial for use in applications such as augmented reality and robotic telesurgery. 4G networks have latency of 30 to 70 ms, with a theoretical lower limit of 10 ms. Early 5G networks have latency as low as 5 ms, with some hoping to reach 1 ms in the future.5
According to Harsh Tataria of Lund University (Lund, Sweden), in a study published in Proceedings of the IEEE, achieving submillisecond latency will require “a complete rethink of the network design, where the present transport networks will begin to disappear and be virtualized over existing fiber, as well as be isolated using modern software-defined networking.” Shortcuts to reduce latency will involve “the reduction or removal of the transport network fiber infrastructure.” Yet even with all wireless links, achieving submillisecond latency would require limiting network reach to the order of a hundred kilometers.
Outlook
We don’t currently know what 6G standards will specify beyond a promise of more bandwidth. Nor can we be sure that 6G will make holographic-quality augmented reality the next big thing.
Wireless data rates need to increase significantly for 6G to deliver applications such as high-resolution virtual reality in the future.
REFERENCES
1. G. P. Fettweis and H. Boche, IEEE Bits: The Information Theory Magazine (Oct. 12, 2021); doi:10.1109/mbits.2021.3118662.
2. See https://bit.ly/3KbPDnU and A. Rogalski and F. Sizov, Opto-electron. Rev., 19, 3, 346–404 (Sep. 2011).
3. H. Haas, L. Yin, Y. Wang, and C. Chen, J. Light. Technol., 34, 6, 1533–1544 (2016).
4. H. Tataria et al., Proc. IEEE, 109, 7, 1166 (Jul. 2021).
5. See https://cnet.co/3FoWqr2.
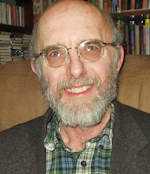
Jeff Hecht | Contributing Editor
Jeff Hecht is a regular contributing editor to Laser Focus World and has been covering the laser industry for 35 years. A prolific book author, Jeff's published works include “Understanding Fiber Optics,” “Understanding Lasers,” “The Laser Guidebook,” and “Beam Weapons: The Next Arms Race.” He also has written books on the histories of lasers and fiber optics, including “City of Light: The Story of Fiber Optics,” and “Beam: The Race to Make the Laser.” Find out more at jeffhecht.com.