Photonic Frontiers: Fiber for Laser Beam Delivery: New fibers deliver the laser beams
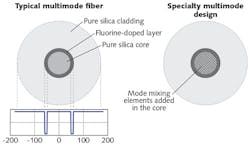
"Fiber optics saved my life," a geologist told me some years back. Optical fibers allowed a physician to perform delicate surgery inside his skull that otherwise would have been impossible. I can't remember the details of his story, but I do recall his delight in being alive to share it with me.
The results of fiber-optic beam delivery are rarely that dramatic, so it's easy to underestimate its importance. Tasks ranging from laparoscopic surgery to welding require precise movement of laser beams. Once, that required bulky articulated arms resembling dental drills. Now, flexible optical fibers can be manipulated easily and precisely by the skilled hands of surgeons in operating rooms or by programmed robotic arms on production lines. New fiber designs promise further improvements.
Power and flexibility
Fiber beam delivery emerged in the 1990s with large-core, step-index multimode fibers delivering light from continuous-wave (CW), high-power diode, and solid-state lasers for industrial applications. A robotic arm could move the tip of the fiber across the workpiece, allowing the laser and the workpiece to remain stationary. Fiber beam delivery was a natural match for fiber lasers when they reached industrial power levels, and is now a standard for many applications using CW output up to the kilowatt range.1
The most widely used beam-delivery fibers have 100 μm cores of pure silica, numerical aperture around 0.22, and outer diameter of 360 μm. A wide range of other fibers are offered for beam delivery, with core diameters from several microns to 1 mm. The challenge now is developing new types able to handle challenging requirements, including delivering ultrafast pulses with high peak powers, or tailoring the delivered beam to match demanding requirements.
Flat-top delivery fiber
At SPIE Photonics West 2016, Clémence Jollivet of Nufern (East Granby, CT) and colleagues described a new type of fiber that scrambles modes to convert a single-mode input beam into a flat-top output beam. Fibers with core diameters of 50, 100, and 200 μm had flat-top output beams with beam parameter products (BPPs) of 2, 4, and 8 mm-mrad.2
Figure 1 shows the flat-top fiber, whose 100 μm silica core contains unidentified mode-mixing elements, and is surrounded by a lower-index, fluorine-doped layer and a pure-silica cladding. Adding the mode-mixing factor may have increased fiber attenuation slightly above pure silica, but loss was below 5 dB/km between 750 and 1330 nm, and was within lot-to-lot variation for pure-silica-core fibers. The fiber could convert the output of a single-mode laser launched into the fiber into a flat-top beam with BPP of 3.8 mm-mrad. When a standard beam-delivery was coupled to the same source, its output had a BPP of 2.6 mm-mrad (see Fig. 2). The flat-top fiber also smoothed the beam profile of a multimode laser source.Nufern is offering the flat-top fibers as a "new technology, not yet as standard products," says Jeff Wojtkiewicz of Nufern. Results have been published, but each fiber must be tailored to produce the desired output from the desired source.
Hollow-core photonic-crystal fibers
The solid-core silica fibers that work well for CW beams are poorly matched to delivering ultrafast pulses with high peak power. The silica is prone to optical damage, has high chromatic dispersion, and suffers strong nonlinearity.
One alternative is beam delivery through hollow glass capillaries, with the interior coated with metal to increase reflectivity. Femtosecond pulse transmission has been demonstrated, but the hollow tubes proved too sensitive to bending losses and too stiff for visible and near-infrared applications.3
Another alternative is using a photonic bandgap to guide light along the core of a hollow photonic-crystal fiber. A 2005 experiment achieved a record-low attenuation of 1.7 dB/km.4 However, about 1% of the core-guided mode overlapped with the cladding, reducing the threshold to laser-induced damage and limiting its use with powerful ultrafast pulses.
Development of hollow photonic-bandgap fibers continues. In addition to potential low loss, they tolerate bending well and can maintain polarization and a single transverse mode in the core. One new type developed by Mattia Michieletto of NKT Photonics (Birkerød, Denmark) and colleagues has core walls structured to be anti-resonant, with modes guided in the core at certain wavelengths.5 They found a fiber containing seven non-touching tubes offered the best combination of suppression of high-order modes with low confinement and bending losses, yielding attenuation of 30 dB/km at 1090 nm. They say more work is needed on fabrication, and hope the future fibers could carry average powers above 100 W through more than 10 km of the fiber.
Others reported encouraging results with hollow-core photonic-crystal fibers at SPIE Photonics West 2016. Björn Wedel and Max Funck of PT Photonic Tools (Berlin, Germany) described microstructured hollow-core fibers that maintained excellent beam quality for ultrafast pulses with hundreds of microjoules of energy and average powers of hundreds of watts. Transmission efficiency exceeded 90% through 3–5 m cables. Dispersion of a few picoseconds per kilometer per nanometer allowed delivery of femtosecond pulses.
"We offer complete beam delivery systems, with the fiber packaged into the system," says Wedel. Input laser pulses are coupled into the fiber through a beam-launching system and connector (see Fig. 3), and then transmitted through up to 10 m of fiber chosen to meet user needs. The fiber can deliver single-mode pulses, which a modular processing head at the end shapes into the desired beam profile. The head can include frequency-doubling optics if needed.Kagome fibers
A microstructure called "kagome" because its ring of hollow cells that forms the characteristic tiling pattern (see Fig. 4) is attracting particular attention. A crucial difference from the usual hollow-core photonic-crystal fiber is that the inner walls of a kagome fiber curve into the center of the fiber rather than away from it. That changes light guidance through the fiber to an inhibited coupling mechanism, reducing overlap of the core mode into the cladding to 0.01%, and gives the fibers a particularly broad spectral range. Gas-filled kagome fibers can compress pulses as well as deliver them, as demonstrated by squeezing a 860 fs, 1030 nm pulse down to 48 fs in experiments at ETH Zurich (Zurich, Switzerland).6Kagome fibers can be made with low loss and vulnerability to laser damage. In 2013, researchers at the XLIM Research Institute (Limoges, France) made 19-cell hypocycloid kagome fibers with record-low attenuation of 17 dB/km at 1064 nm.3 They consider those properties very attractive for delivering ultrafast laser pulses.
The Limoges group collaborated with Sebastian Pricking and coworkers at Trumpf Laser (Schramberg, Germany) to transmit single-mode sub-picosecond pulses at 1030 nm through an air-filled hypocycloid kagome fiber. At SPIE Photonics West 2015, they reported loss of 20 dB/km and 80% transmission efficiency with an M2 parameter of 1.15.7 At SPIE Photonics West 2016, Pricking and colleagues reported integrating mode-matching and coupling optics into the laser head, connecting the fiber via LLK-D connectors, and patented hermetical sealing of the fiber within a flexible hose. They reported transmitting pulses up to 500 mJ at average power up to 150 W through a 5 m fiber. Depending on the fiber length, they obtained overall efficiency above 85% and M2<1.3 beam quality. Perhaps most impressively, they maintained robust coupling into the fiber over weeks of operation without active beam steering.8
A spinoff from the Limoges group, GLOphotonics (Limoges, France) manufactures both kagome (inhibiting coupling guiding) and band-gap guiding hollow photonic-crystal fibers. Their standard products include 7-cell hollow photonic-crystal fibers and 1-, 7-, and 19-cell kagome fibers, with attenuation of 50 dB/km in the 7-cell fibers designed to transmit 1030 nm. Fetah Benabid, a group leader at XLIM and an advisor at GLOphotonics, described their work in detail in our September 2014 issue.9
Beam delivery in other bands
Kagome fibers have also been demonstrated at terahertz frequencies, where they have attenuation well below material losses.10
Hollow-core glass capillaries with interior metal/dielectric structures can transmit infrared wavelengths beyond the silica transmission range. Polymicro Technologies (Phoenix, AZ) coats the interiors of capillaries with 500–1000 μm bores with silver and silver iodide layers that can be optimized to transmit 10 μm carbon-dioxide (CO2) beams for conventional and laparoscopic surgery. Different hollow fibers are available for the 2.94 μm erbium-YAG laser line, also used in surgery. Biocompatible jackets can withstand sterilization for invasive surgery.
Single-mode transmission has been possible in single-mode bores no more than 40 times the wavelength for the CO2 band and longer. Now, Angelo Sampaolo and colleagues at the University of Bari Also Moro (Bari, Italy) have demonstrated single-mode transmission at 5.11–10.5 μm through flexible hollow waveguides with 200 μm bores up to 50 cm long.11 That extends coverage to much of the range of quantum cascade lasers.
Other new twists are being tried in solid-core fibers. Jayanta Sahu's group at the University of Southampton (Southampton, Hampshire, England) is studying a new type of fiber with multiple thin low-index "trenches" surrounding the core for use in the visible and near-ultraviolet. A key attraction is that multi-trench fibers can be fabricated by modified chemical vapor deposition, making them suitable for mass production. The fibers strongly attenuate higher order modes, allowing effective single-mode transmission at 405 nm with a large effective area of 10 μm core. The authors say the big advantage of multi-trench fibers is their easier splicing and processing than photonic-crystal fibers.12
Outlook
Fiber beam delivery offers important advantages for many applications. The big challenge is to expand it, particularly into the growing field of ultrafast pulse applications, which put more strain on the delivery fiber because of their high peak intensity. We're also seeing increasing specialization, with new fibers being developed to deliver particular beam profiles. Medical applications will require more packaging innovations to make biocompatible beam-delivery systems. So I won't be surprised the next time somebody tells me fiber optics saved their life.
REFERENCES
1. B. Wedel and M. Funck, "Industrial fiber beam delivery enhances ultrafast laser machining," Industrial Laser Solutions, 31, 1, 28–30 (Jan/Feb 2016).
2. C. Jollivet et al., "Specialty flat-top beam delivery fibers with controlled beam parameter product," Proc. SPIE, 9727, Laser Resonators, Microresonators, and Beam Control XVIII, 97270T (Mar. 8, 2016); doi:10.1117/12.2209348.
3. B. Debord et al., Opt. Express, 21, 23, 28597 (Nov. 18, 2013); doi:10.1364/oe.21.028597.
4. P. J. Roberts et al., Opt. Express, 13, 1, 236–244 (2005).
5. M. Michieletto et al., "High-power picosecond pulse delivery through hollow core photonic band gap fibers," Proc. SPIE, 9728, Fiber Lasers XIII: Technology, Systems, and Applications, 97282Z (Mar. 11, 2016); doi:10.1117/12.2211324.
6. F. Emaury et al., Opt. Express, 21, 4, 4986–4994 (2013); doi:10.1364/oe.21.004986.
7. S. Pricking et al., "Hollow core fiber delivery of sub-ps pulses from a TruMicro 5000 Femto edition thin disk amplifier," Proc. SPIE, 9356, High-Power Laser Materials Processing: Lasers, Beam Delivery, Diagnostics, and Applications IV, 935602 (Mar. 9, 2015); doi:10.1117/12.2079289.
8. S. Pricking et al., "Industrial grade fiber-coupled laser systems delivering ultrashort high-power pulses for micromachining," Proc. SPIE, 9741, High-Power Laser Materials Processing: Lasers, Beam Delivery, Diagnostics, and Applications V, 974109 (Mar. 18, 2016); doi:10.1117/12.2213356.
9. F. Benabid et al., "Kagome PC fiber goes to extremes in ultrashort-pulse lasers," Laser Focus World, 50, 9, 29–34 (Sep. 2014).
10. J. Anthony et al., "Air-core microstructured fibers provide low-loss, broadband terahertz guidance," Laser Focus World, 48, 3 (Mar. 2012).
11. A. Sampaolo et al., Opt. Express, 23, 1, 195–204 (2015); doi:10.1364/oe.23.000195.
12. D. Jain et al., Opt. Lett., 40, 5026 (Nov. 1, 2015).
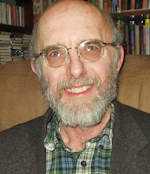
Jeff Hecht | Contributing Editor
Jeff Hecht is a regular contributing editor to Laser Focus World and has been covering the laser industry for 35 years. A prolific book author, Jeff's published works include “Understanding Fiber Optics,” “Understanding Lasers,” “The Laser Guidebook,” and “Beam Weapons: The Next Arms Race.” He also has written books on the histories of lasers and fiber optics, including “City of Light: The Story of Fiber Optics,” and “Beam: The Race to Make the Laser.” Find out more at jeffhecht.com.