Photonic Frontiers: Fiber Optic Communications: Pushing fiber line rates beyond 100 Gbit/s
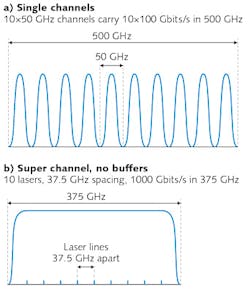
Coherent transmission at 100 Gbit/s has become standard in the global fiber-optic backbone network, and more is coming. A few systems are operating at hundreds of gigabits, and hero experiments have exceeded a petabit (1015 bits/s) over single developmental fibers that can transmit light on dozens of separate paths. It may remind you of the explosive growth of the 1990s.
Yet today's picture is more complex because the technology is advancing on three fronts—existing systems based on step-index "standard" singlemode fiber, new systems using large mode-area fibers, and development of spatial-division multiplexing over new fiber types
Coherent transmission and digital signal processing are making the most of the 9-μm-core singlemode fiber used since the 1980s. Dark fibers installed during the bubble years remain widely available on North American and European routes. Today's long-haul coherent systems can transport 100 Gbit/s signals on close to a hundred 50 GHz optical channels on such fibers—a total of 10 Tbit/s per fiber pair—and new technology may offer some further increase.
Large mode-area fibers are preferred for new submarine and terrestrial cables. Their low nonlinearity allows them to carry higher data rates over longer distances.
In the longer term, developers are working on new fiber types that can multiply capacity by spatial-division multiplexing-using separate cores within a fiber and separate modes within few-mode cores. Potential applications include long-haul transmission and shorter distances, from within server farms to metro distribution networks.
Approaching the wall with standard singlemode fiber
Today's 10 Tbit/s capacity represents a factor-of-25,000 increase since standard singlemode fibers began carrying 400 Mbit/s in the mid-1980s. Ganging coherent transmitters together to form "superchannels" in multiples of 100 Gbit/s can increase capacity about another 30%. The trick is to combine signals from several laser transmitters, eliminating the buffer zones that separate traditional 50 GHz channels (see Fig. 1).
Demonstrated single-channel data rates have reached the terabit level. In 2014, Infinera (Sunnyvale, CA) sent a 1 Tbit/s superchannel signal 500 km through a loop of installed fiber between Budapest, Hungary and Bratislava, Slovak Republic. A single photonic integrated circuit contained the 10 laser sources. The prototype 1 Tbit/s line card can cover much longer spans using polarization-multiplexed quadrature phase-shift keying (PM-QPSK), says Geoff Bennett of Infinera. Using current production-level 500 Gbit/s line cards, Infinera and Facebook spanned 4000 km without regeneration. Bennett credited the improvement to splitting signal processing between transmitter and receiver in second-generation coherent systems.
However, standard singlemode fiber is approaching the nonlinear version of the Shannon limit on error-free transmission capacity.1 Noise imposes the traditional Shannon limit, so capacity of a linear medium can be increased by complex coding schemes that generate higher power to increase signal-to-noise ratio. However, optical fiber is a nonlinear medium, so the extra power from complex coding generates nonlinear noise. This decreases the signal-to-noise ratio and thus imposes a more stringent limit on transmission efficiency, usually measured as bits per second of signal per hertz of bandwidth. The result is an inherent tradeoff between coding rate and transmission range in a fiber (see Fig. 2).Large-mode-area fibers carry more data
Large-mode-area fibers spread signals over a larger area, reducing power density and nonlinear noise. That extends transmission capacity and reach, so solid-core large-area fibers have become standard for new long-haul terrestrial and submarine cables. Both Corning (Corning, NY) and OFS (Norcross, GA) offer fibers with effective mode areas of at least 125 μm2 and attenuation below 0.19 dB/km in the 1.55 μm band. Effective mode areas have reached over 1000 μm2 in photonic-crystal fibers, but their loss is far too high for use in communications.
Large-mode-area singlemode fibers usually have a high chromatic dispersion. But that's no longer a problem because coherent transmission and digital signal processing have enabled powerful electronic dispersion compensation.
The spread of large-area fibers allows use of higher power and advanced coding techniques to increase data rates and distances. Ciena (Hanover, MD) sent a 1 Tbit/s superchannel with 16-QAM modulation carrying live traffic through nearly 1000 km of such fiber in the Comcast (Philadelphia, PA) long-haul network. Thanks to the advanced coding scheme, the spectral efficiency reached 5 bits/s/Hz. Bennett says that laboratory tests of low-mode-area fibers stretched the reach of PM-8QAM signals nearly a factor of three to transatlantic distances.
Large-area, low-loss fibers also have become standard for long-haul submarine cables, as Neal Bergano of TE Connectivity SubCom (Eatontown, NJ) mentions in this month's Future Optics interview (see page 21). Transoceanic cables with eight fiber pairs and advanced coding have capacity of 80 Tbits. In a recent experiment, Bergano's group transmitted 152 200 Gbit/s polarization-multiplexed 16-QAM channels with efficiency of 6 b/s/Hz through a 9748 km testbed.2 But capacity is expected to top out about a factor of 10 above standard singlemode fiber.
Spatial-division multiplexing
For the long term, spatial-division multiplexing offers a potential hundred-fold capacity boost by sending signals on parallel physical routes between two points. Peter Winzer of Bell Labs calls it the fifth physical dimension of optical multiplexing, after time, phase, frequency, and polarization.3
Spatial multiplexing through separate fibers in the same cable is well established, but offers little prospect for integration of other components such as amplifiers. Multicore fibers and multiple-mode cores offer much more hope for integration, but they require extensive development. "We all know that parallel systems will happen. The question is which form of parallelism makes the most economic sense," says Winzer.
Early multimode and multicore tests were encouraging. At the 2012 European Conference on Optical Communications (ECOC), Nippon Telegraph and Telephone (Tokyo, Japan) sent a record 1010 Tbit/s (1.01 Pbit/s) through 52.4 km of a 12-core fiber.4 Each core carried 380 Gbit/s on 222 separate wavelengths, a total of 84.5 Tbits per core. Modal-division multiplexing was demonstrated separately on few-mode fibers.
At last year's Optical Fiber Communications (OFC 2015) conference, the postdeadline hero experiments session heard three reports of multimode transmission in each of several cores in multicore fibers. Two described spatial division multiplexing through more than 100 paths on several kilometers of fiber. J. Sakaguchi of the National Institute of Information and Communications Technology (Tokyo, Japan) sent three modes through each of 36 cores in their 5.5 km fiber (see Fig. 3),5 and Koji Igarashi of KDDI R&D Labs (Saitama, Japan) sent six modes through each of 19 cores of a 9.8-km fiber.6 In a third paper, Kouki Shibahara and colleagues at NTT Laboratories reported sending signals in three modes on each of 12 cores 10 times through a 52.7 km loop with an amplification stage. Demonstrating amplification, transfer of signals between fibers, and reaching a total distance of more than 500 km were important steps, although the NTT group used fewer levels of spatial multiplexing.7Big questions remain. How much crosstalk will occur between modes in few-mode fibers during coupling and during amplification? How well can amplifiers and couplers be integrated? How much can spatial-division multiplexing increase efficiency measured in bits per second per hertz? How much spatial-division multiplexing will be possible over transoceanic distances?
Recent theoretical work hints at unexpected limitations. At the 2015 ECOC, Kasyapa Balemarthy and Robert Lingle of OFS warned that a 220 μm fiber could accommodate no more than 5–7 cores without degrading 100 Gbit/s signals after 6000–12,000 km.8
Outlook
The bottom line question is what technology offers the best value. The main attraction of integrating spatial division multiplexing is the potential to lower costs. Yet, as Winzer says, "Nobody can build a 19-core fiber at a lower cost than 19 individual fibers." So at best, multicore, multi-mode fiber is years from practicality.
Yet if you look back, in the late 1970s many people thought it would be more practical to lay many parallel multimode fibers than one singlemode fiber. In the 1980s, coherent transmission was deemed impractical, and would stay on the shelf for two decades before new technology opened the door to 100 Gbit/s coherent transmission. Other options also remain, including expanding the transmission spectrum beyond the 1530 to 1565 nm erbium-fiber C band. And stay tuned for any surprises at the OFC 2016 postdeadline sessions on March 24.
REFERENCES
1. A. D. Ellis et al., "The nonlinear Shannon limit and the need for new fibres," Proc. SPIE, 8434, 84340H (Jun. 1, 2012); doi:10.1117/12.928093.
2. J.-X. Cai et al., Opt. Express, 22, 4, 9115 (Apr. 8, 2014); doi:10.1364/oe.22.009116.
3. P. Winzer, Bell Labs Techn. J., 19, 22 (2014); doi:10.15325/bltj.2014.2347431.
4. H. Takara et al., "1.01-Pb/s (12 SDM/222 WDM/456 Gb/s) crosstalk-managed transmission with 91.4-b/s/Hz aggregate spectral efficiency," Proc. ECOC, Th.3.C.1 (Sept. 2012).
5. J. Sakaguchi et al., "Realizing a 36-core, 3-mode fiber with 108 spatial channels," Proc. OFC, Th5C.2 (2015).
6. K. Igarashi et al., "114 space-division-multiplexed transmission over 9.8-km weakly-coupled-6-mode uncoupled 19-core-fibers," Proc. OFC, Th5c.4 (2015).
7. K. Shibahara et al., "Dense SDM (12 core × 3 mode) transmission over 527 km with 33.2-ns mode-dispersion employing low-complexity parallel MIMO frequency domain equalization," Proc. OFC, Th5C.3 (2015).
8. K. Balemarthy and R. Lingle Jr., "Upper limits on number of cores for multi-core SMFs over trans-oceanic distances at 100Gbps," Proc. ECOC, 0761 (2015).
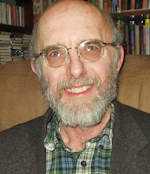
Jeff Hecht | Contributing Editor
Jeff Hecht is a regular contributing editor to Laser Focus World and has been covering the laser industry for 35 years. A prolific book author, Jeff's published works include “Understanding Fiber Optics,” “Understanding Lasers,” “The Laser Guidebook,” and “Beam Weapons: The Next Arms Race.” He also has written books on the histories of lasers and fiber optics, including “City of Light: The Story of Fiber Optics,” and “Beam: The Race to Make the Laser.” Find out more at jeffhecht.com.