Specialty Fibers: Suspended-core fiber with sensing nanoparticles could benefit healthcare
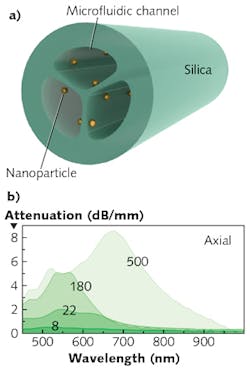
An optical fiber that is designed for sensing contains either discrete or continuous light-based sensing elements somewhere along the fiber. When the fiber is immersed in the environment to be sensed, light is used to query the sensing elements and thus characterize the environment along the path of the fiber. Common uses include the measurement of temperature, pressure, and/or strain. The fiber-sensing technologies vary widely, as do the environments to be sensed, which include building and bridge structures, wind-turbine blades, downhole portions of oil and gas wells, and many others.
One interesting, and growing, area of application for fiber sensing is in the medical and life-sciences arena. Here, sensing lengths are on the order of millimeters to meters, rather than the kilometers-long fiber sections often used, for example, in the oil and gas industry. Sometimes, only a single property needs to be measured.
The noninvasive detection of disease is a burgeoning area of research in biophotonics. One light-based noninvasive detection technique relies on the use of metallic nanoparticles that, when excited by light, produce plasmon resonances that can change in wavelength in the presence of the substance to be sensed, serving, for example, as a refractive-index-change sensor. This technique can be adapted to fiber-based sensing.
These nanoparticles can be attached to the outside of optical fibers that have diameters small enough that their evanescent-wave region extends past the outer surface of the fiber into the surrounding analyte, allowing light carried by the fiber to excite the plasmonic nanoparticles. However, this approach results in a very delicate fiber probe that can easily lose its nanoparticles. In addition, this type of fiber sensor senses only a very small percentage of the volume of the surrounding analyte.
Suspended-core fiber
An alternative approach to fiber sensing with attached plasmonic nanoparticles developed by scientists at the Leibniz Institute of Photonic Technology and Friedrich-Schiller-University (both in Jena, Germany) is based on the use of a silica suspended-core fiber (SCF), with the nanoparticles attached to the outside of the fiber's core and protected by the fiber's surrounding cladding (see figure).1
In the SCF geometry, a few longitudinal open channels are configured in a pie-slice arrangement around the fiber's axis. The channels are separated by very thin silica webs that meet in the center to form a tiny solid core. Because the core is so small, the evanescent field of the light it carries extends far beyond the core and is thus able to excite plasmonic structures attached to the core or otherwise located nearby.
The experimental fiber contains three channels, each with a core-to-channel-edge size of about 30 μm. The three 0.5-μm-thick silica webs combine at the fiber's center to form a solid core with a diameter of about 2.8 μm. The fiber was fabricated by inserting three capillaries into a silica jacketing tube and drawing the combined structure down into fiber.
The researchers chemically functionalized the inside surfaces of the fiber channels, then drew a suspension of gold 34-nm-diameter nanospheres into the fiber using a peristaltic pump. Sections of fiber up to 6 m in length were filled with the suspension, resulting in a homogeneous nanoparticle density along the fiber. Fibers with different densities of nanoparticles were fabricated for comparison.
In the experimental optical setup, two measurement configurations for the measurement section were used. One, comprising only a section of nanoparticle-containing SCF (NP-SCF) fiber, allowed the determination of modal attenuation, while the second, containing a light-delivering section of SCF that was connected to a NP-SCF fiber section, was used for determining sensitivity to refractive index.
Broadband light (450 nm to 2.4 μm) from a supercontinuum source made by NKT Photonics (Birkerød, Denmark) was launched into the fiber core using a microscope objective with a numerical aperture (NA) of 0.9. At the fiber's other end, the output mode was either coupled to an optical spectrum analyzer (OSA) or imaged onto a camera with selectable narrowband optical filters.
For modal-attenuation measurements, the fiber's channels were filled with air and the fiber repeatedly cut to shorter and shorter lengths (the removal of the fluid and subsequent high scattering because of the air path meant that short sections from 1 cm down to 2 mm were measured). For the measurements of refractive-index sensitivity, the researchers took sections of NP-SCF and flushed them with a number of different refractive-index oils from Cargille Laboratories (Cedar Grove, NJ) via a syringe pump until no air bubbles were seen.
Viable with low nanoparticle densities
Spectral measurements for different particle densities showed that a higher density of the nanoparticles produced a higher modal attenuation, as expected. However, the very small core diameter allowed very low nanoparticle densities of down to 2 NP/μm2, as well as high densities up to 500 NP/μm2. The spectral curves showed a large increases near the bulk localized surface plasmon resonance (LSPR) wavelength.
For the highest particle densities, the nanoparticles were so close together that they began to interact with each other as well as with the surroundings, as seen by the experimental departure from the theoretical linear relationship between particle density and modal attenuation.
Measurements of refractive-index sensitivity showed that the wavelength of the LSPR spectral dip moved to longer wavelengths as the refractive index increased. The resulting spectral refractive-index sensitivity was 167 nm/RIU (RIU, or refractive-index unit, is merely a way of allowing refractive index, which is actually dimensionless, to be used as a dimensional term).
The researchers are especially interested in seeing this noninvasive bioanalytic technique applied in the areas of molecular-disease diagnostics and environmental science.
REFERENCE
1. B. Doherty et al., Biomed. Opt. Express (Feb. 1, 2017); http://dx.doi.org/10.1364/boe.8.000790.
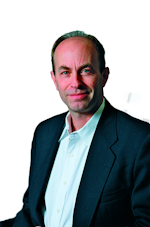
John Wallace | Senior Technical Editor (1998-2022)
John Wallace was with Laser Focus World for nearly 25 years, retiring in late June 2022. He obtained a bachelor's degree in mechanical engineering and physics at Rutgers University and a master's in optical engineering at the University of Rochester. Before becoming an editor, John worked as an engineer at RCA, Exxon, Eastman Kodak, and GCA Corporation.