NEXT-GEN COMMUNICATIONS FIBER: Multilevel modulation formats push capacities beyond 100 Gbit/s
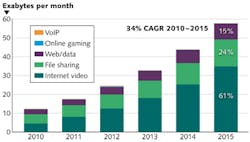
ABHAY M. JOSHI, SHUBHASHISH DATTA, and ANDREW CRAWFORD
Since the late 1980s, fiber-optic networks have steadily become the bedrock for the ever-expanding global telecommunications system. Early fiber-optic links, such as the eighth transatlantic telecommunications cable (TAT-8) installed in 1988, were relatively simple systems by today’s standards and used on-off signaling to transmit a few hundred megabits per second (Mbit/s) over a single optical fiber.
The year 1992 saw the deployment of wavelength-division multiplexing (WDM) that allowed multiple optical channels, each allocated a unique optical carrier frequency, to co-propagate over the same optical fiber. Coupled with innovations in optical amplification, WDM triggered an exponential growth in information capacity of a single optical fiber and heralded the “Information Age.”
Modern WDM networks, incorporating as many as 80 optical channels with 50 GHz grid spacing, have reached the spectral limit of optical fiber amplifiers used in these systems. As a result, further expansion of information capacity through incremental “horizontal stacking” of optical channels is not feasible. However, the global demand for information, primarily fueled by video traffic, is expected to continue its growth (see Fig. 1).1Coherent detection of high-level modulation formats, supplemented by WDM, has lately emerged as the solution to further upgrade the fiber-optic backbone networks.
High-level modulation formats
Information channels fundamentally transport binary data streams between various signal-processing systems incorporating binary electronic logic circuits. Efficient transmission of information over a physical medium invariably involves aggregating multiple binary streams in independent orthogonal dimensions.
Wavelength-division multiplexing exploited one such dimension, namely optical carrier frequency, to allow aggregation of multiple optical channels. Each individual optical channel has so far used binary signaling schemes, such as on-off keying (OOK) or differential phase-shift keying (DPSK), having a spectral efficiency of 1 bit/symbol. Consequently, the information throughput of a single optical channel has been progressively enhanced to 40 Gbit/s by increasing the corresponding symbol rate and the analog bandwidths of optoelectronic and electronic subsystems.
Given the current WDM grid spacing of 50 GHz, it is difficult to further increase the symbol rate to, say, 100 Gbaud, while avoiding crosstalk between optical channels. Therefore, it has become necessary to utilize other orthogonal dimensions, namely optical phase and optical polarization, through high-level modulation to enhance the information throughput without increasing the symbol rate (see Fig. 2).Exploiting optical phase allows multiplexing two binary data streams, in-phase (I) and in quadrature (Q) to the same optical carrier, as demonstrated by the signal constellation for quadrature phase-shift keying (QPSK). Two such QPSK signals can be independently modulated on orthogonal optical polarization states, resulting in a spectral efficiency of 4 bits/symbol. In fact, the telecommunications industry has agreed to utilize dual-polarization QPSK (DP-QPSK) for upgrading optical channels to 100 Gbit/s with a symbol rate of 25 Gbaud.2
Further increase in spectral efficiency will require combining multiple binary data streams on a single orthogonal dimension; that is, a combination of optical carrier frequency, phase, and polarization, as is the case with dual-polarization 16-quadrature amplitude modulation (DP-16QAM). More complicated modulation schemes with very high spectral efficiency, such as DP-64QAM, are also being investigated.3 Unfortunately, enhancing the dimensionality of the symbols increases the complexity of the transceiver design.
DP I-Q optical transmitter
Imposing a binary modulation format, OOK or DPSK, on an optical carrier requires only one Mach-Zehnder modulator (MZM) in the optical transmitter. Exploiting both optical phase and polarization necessitates four MZMs—one for each orthogonal dimension. Each MZM impinges the input electrical signal on one polarization state and in-phase to the transmitter laser signal (optical carrier). Two optical phase shifters and a polarization rotator are needed to transfer the relevant binary data streams into the other three dimensions (see Fig. 3). For modulation formats with higher spectral efficiency than DP-QPSK, digital-to-analog converters (DACs) are also needed to aggregate multiple electrical binary signals prior to optical modulation.Coherent DP I-Q optical receiver
Variations in optical intensity can be directly detected by a photoreceiver of sufficient bandwidth, as is the case with OOK optical receivers. In contrast, optical phase and polarization can only be defined with respect to a reference optical signal. Therefore, deciphering high-order modulation formats requires mixing the received signal with an optical local oscillator (LO) in a coherent receiver.
In addition, coherent detection results in a linear transfer function between the received optical field and the electrical output. This allows correcting for linear fiber propagation impairments such as chromatic dispersion and polarization-mode dispersion in the electronic domain using ultrafast analog-to-digital converters (ADCs) integrated with digital signal-processing (DSP) chips (see Fig. 4). Unlike the DP I-Q transmitter, no additional receiver components are needed for detecting higher-level modulation formats, such as DP-16QAM.Challenges
Generation and reception of optical signals with high-level modulation formats substantially increases the component count in the transceiver design, raising concerns about size constraints. Also, multiplexing and demultiplexing four optical orthogonal dimensions requires careful consideration of amplitude and phase symmetry between the parallel signal paths.
But these issues can be reasonably addressed through photonic integration, as envisioned by the Optical Internetworking Forum (OIF; Fremont, CA)—a consortium of member companies that promotes the development and deployment of interoperable communications network solutions through Implementation Agreements (IAs).4,5 Photonic integration also shows the promise of reducing manufacturing costs incurred during optical packaging of the transceivers’ subsystems.
Inclusion of ADC and DSP in the coherent receiver adds another technical challenge; namely, dynamic range. Optical receivers for binary OOK and DPSK formats require an essentially high-speed comparator, implemented in clock and data recovery (CDR) chips, to convert the photoreceivers’ electrical analog output into the electronic digital domain.
One would imagine that four such CDR circuits would suffice in a DP-QPSK coherent receiver, where each optically deconstructed orthogonal axis should ideally contain binary (1 bit) information. But due to lack of a polarization stabilizer and an optically phaselocked local oscillator in the receiver design, the four electrical analog outputs of the balanced photoreceiver array are significantly cross-coupled, and are separated only in the digital domain.
Consequently, it is necessary to quantize the electrical analog signals with effective number of bits (ENOBS), assuming that forward error correction (FEC) can convert a bit-error-ratio (BER) of 1 × 10-3 to an essentially error-free performance. This overhead of approximately 3 bits is expected to hold for higher-order modulation formats, such as DP-16QAM and DP-64QAM.6 Combined with concerns over power dissipation and synchronization, achieving such dynamic range or ENOBS in the ADC and DSP is not trivial.
Recent advances provide a solution for 25 Gbaud (100 Gbit/s) DP-QPSK transmission: 40 nm complementary metal-oxide semiconductor (CMOS) application-specific integrated circuits (ASICs) incorporating both ADC and DSP functionality.7 Higher-order modulation formats will require further improvements in ADCs as well as similar performance for the DACs required for the transmitter.
Increasing the information capacity of optical WDM links, while maintaining the current 50 GHz grid spacing, requires increasing the spectral efficiency of the modulation format. Optical coherent detection of high-order modulation formats exploits the hitherto unused parameters of optical phase and polarization to upgrade the information capacity of optical channels to 100 Gbit/s and beyond. Enabled by significant advances in photonic integration and ultrafast electronic processing, these upgraded links are expected to fuel the continuing growth of global telecommunications.
REFERENCES
1. Cisco white paper, “Broadband Access in the 21st Century: Applications, Services, and Technologies,” www.cisco.com/en/US/solutions/collateral/ns341/ns525/white_paper_c11-690395.pdf (2011).
2. Optical Internetworking Forum Implementation Agreement on “100G Ultra Long Haul DWDM Framework Document,” OIF-FD-100G-DWDM-01.0 (June 2009).
3. F. Buchali, “Technologies towards Terabit Transmission Systems,” 2010 European Conference on Optical Communication (ECOC), tutorial paper We.6.C.1, Turin, Italy (2010).
4. Optical Internetworking Forum Implementation Agreement on “Implementation Agreement for Integrated Polarization Multiplexed Quadrature Modulated Transmitters,” OIF-PMQ-TX-01.0 (March 2010).
5. Optical Internetworking Forum Implementation Agreement on “Implementation Agreement for Integrated Dual Polarization Intradyne Coherent Receivers,” OIF-DPC-RX-01.1 (September 2011).
6. T. Pfau, S. Hoffmann, and R. Noe, J. Light-wave Technol., 27, 989–999 (2009).
7. I. Dedic, “High Speed CMOS DSP and Data Converters,” 2011 Optical Fiber Communication Conference (OFC), paper OTuN1, Los Angeles, CA (2011).
Abhay M. Joshi is president and CEO, Shubhashish Datta is a photonics engineer, and Andrew Crawford is a systems engineer at Discovery Semiconductors, 119 Silvia St., Ewing, NJ 08628; e-mail: [email protected]; www.discoverysemi.com.